the Creative Commons Attribution 4.0 License.
the Creative Commons Attribution 4.0 License.
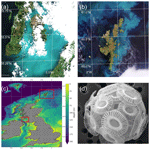
Unusual coccolithophore blooms in Scottish waters
Richard Renshaw
Eileen Bresnan
Susan Kay
Robert McEwan
Peter I. Miller
Paul Tett
Two unusual blooms were observed in Scottish waters during summer 2021: one in the Clyde Sea and the other by the east coast of the Shetland Islands. Both had the appearance of coccolithophore blooms. Transmission electron microscopy of a sample from the Clyde Sea confirmed the presence there of the coccolithophore Emiliania huxleyi. We examine the conditions that led to these unusual blooms. In situ data are scarce, and so we draw inference from satellite data and reanalysis. For Shetland, the bloom can be seen to originate further north on the edge of the continental shelf. It is advected south and then west towards the Shetland coast by surface currents. For the Clyde Sea region, April 2021 was the coldest April of the last 30 years (National Climate Information Centre). We hypothesise that this cold weather restricted the usual spring bloom of diatoms. A restricted spring bloom would mean higher-than-usual concentrations of nutrients in the summer. It might also mean reduced numbers of grazers. These factors would provide ideal conditions for coccolithophores to flourish as temperatures and sunlight increase.
- Article
(7244 KB) - Full-text XML
- BibTeX
- EndNote
Coccolithophores belong to a diverse group of phytoplankters (class Prymnesiophyceae) that is widespread in the oceans. The ecological group that includes Emiliana is particularly abundant in upwelling and temperate sub-polar regions (Balch et al., 2019). Most coccolithophores are not themselves harmful or toxic, but they are of ecological importance, particularly for carbon cycling and sequestration (Rost and Riebesell, 2004). They typically produce an exoskeleton consisting of several calcium carbonate plates called coccoliths (Young et al., 1999). These coccoliths are not opaque (phytoplankton require light for photosynthesis), but they scatter and polarise light. Coccolith shedding occurs during the later stages of the bloom life cycle, when the cells are threatened, for example by pathogen pressure (Frada et al., 2008). During this stage, the coccoliths are shed and accumulate in the surrounding water. The visual effect is to turn the sea a milky turquoise colour, visible to the human eye and in satellite imagery.
The function of the coccoliths is unclear. They are believed to be protective, either against grazing, against viral or bacterial attack, or as a refractor of light that acts as a sunshade in excessively bright conditions (Monteiro et al., 2016). Johns et al. (2023) show that coccoliths initially provide some protection from viral attack but once shed can mediate such attacks. Müller (2019) suggests that coccolith production may have evolved originally as an efficient mechanism for intracellular Ca2+ detoxification at a time of elevated seawater Ca2+ concentrations (e.g. during the Cretaceous and Jurassic periods).
Summer 2021 saw milky, turquoise-coloured waters caused by algal blooms in two locations off Scotland, in the Clyde Sea on the west coast and also to the east of the Shetland Islands (Fig. 1). These blooms were visually striking and so unusual that they were reported in the news (e.g. Bradshaw, 2021). Transmission-electron-microscope analysis of a water sample from the Clyde Sea (Fig. 1d) confirmed the algae to be a morphotype of coccolithophore, Emiliania huxleyi (morphotype B). Blooms of this organism are common in spring and early summer in the North Atlantic and occur in some years in the northern North Sea and the western English Channel. However, such striking occurrences have not been reported from the Clyde Sea for many years. Colleagues of one of the authors (PT) recall no such event since 1983, when they remember sampling turquoise waters and coccolithophores in sea lochs of the Firth of Clyde. In this paper we use observations and reanalysis data to look for unusual environmental conditions in 2021 that might have allowed these blooms to thrive.
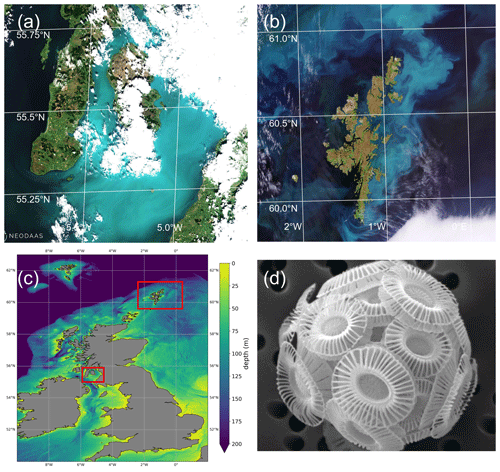
Figure 1(a) Sentinel-2 MSI image of Clyde Sea on 21 June 2021 at 11:35 UTC, true colour with enhanced contrast. Processed by the Natural Environment Research Council Earth Observation Data Acquisition and Analysis Service (NEODAAS) using ACOLITE atmospheric correction. (b) Sentinel-2 MSI image of Shetland Islands on 1 July 2021, processed by ESA (https://www.esa.int/ESA_Multimedia/Images, last access: 30 May 2023). (c) Bathymetry map with locations of (a) and (b) marked in red. Shelf edge is visible as transition from light blue (less than 200 m depth) to dark blue (deep water). (d) Scanning electron micrograph of sample from the Clyde Sea, June 2021, identified as E. huxleyi, morphotype B. Credit: Eileen Bresnan (Marine Scotland Science).
2.1 Ocean colour
Ocean colour (OC) instruments measure water-leaving radiation at various wavelengths in the visible and near-infrared spectrum. Satellite measurements of ocean colour are used here in two forms. One is imagery. The enhanced colour maps from Sentinel-3 OLCI (Ocean and Land Colour Imager) and Sentinel-2 MSI (Multi-Spectral Imager) in Fig. 2 provide visual indications of algal blooms. The other is observation products, point estimates of near-surface chlorophyll concentration derived from multiple sensors. The CMEMS (Copernicus Marine Environment Monitoring Service) product used here (product ref. no. 2, Table 1) estimates chlorophyll concentration for several distinct phytoplankton functional types, including diatoms and nanophytoplankton. The nanophytoplankton category includes E. huxleyi.
Several factors make chlorophyll estimation difficult for coastal waters. Bowers et al. (2000) find that dissolved organic matter (DOM) from fresh water is usually the largest optically active constituent in the Clyde Sea. Ocean colour algorithms are designed to minimise errors due to suspended sediment and DOM. The presence of large numbers of coccoliths would also have a strong impact on backscattered radiation (Voss et al., 1998). The estimation process does mask for cloud, sun glint, and coccoliths (OC-CCI, 2020). This masking means there are few OC chlorophyll estimates available for the Clyde Sea during the period of the Clyde bloom (12 June to 7 July 2021). We use the OC product in Fig. 5 not as a measure of absolute value but to show the timing of growth and also how values in 2021 compare to other years.
2.2 North West Shelf reanalysis
The North West Shelf (NWS) reanalysis (product ref. no. 1, Table 1) is based on the physical ocean model NEMO (Madec and Team, 2008) at 7 km horizontal resolution and 51 vertical levels, with tides represented, over a domain that encompasses the North West Shelf (Renshaw et al., 2021). Atmospheric forcing is from the ERA5 atmospheric reanalysis (Hersbach et al., 2023). River discharge volumes for the year 2018 onwards come from a daily climatology. The reanalysis uses NEMOVAR (Mogensen et al., 2012) to assimilate observations of physical variables (satellite sea surface temperature, SST, as well as in situ temperature and salinity profiles; Waters et al., 2015).
Here we use the reanalysis for sea surface temperature and also for near-surface currents in the region of the European Slope Current. Reanalysis SST is strongly constrained by high-quality satellite observations. Variations in surface currents are driven predominantly by surface winds (Röhrs et al., 2023), provided here by ERA5. The slope current itself is forced also by meridional density gradient and steep bathymetry (Marsh et al., 2017). Validation of the reanalysis shows that it does produce a realistic slope current (Renshaw et al., 2021).
Figure 1 shows a bloom on the eastern side of Shetland. There is no information on the species present, but the brightness and turquoise colour of the bloom suggest coccolithophores. Coccolithophore blooms in the North Sea are not unusual. An unusual feature of the 2021 bloom was that it came so close inshore. Examination of imagery for the years 2017 to 2020 from Plymouth Marine Laboratory (PML) (described in Highfield et al., 2014) and of a dataset of coccolithophore blooms for 1998 to 2016 in Kondrik et al. (2019) finds no other examples where blooms intrude among the islands and bays on the eastern side of Shetland.
Kondrik et al. (2019) show that often blooms develop further south and east in the North Sea in spring or early summer and are advected by an anti-clockwise circulation sometimes reaching as far north as Shetland. Sometimes a bloom originates in the north, along the northern edge of the continental shelf, and is advected southwards. Bathymetry in Fig. 1c shows the location of this shelf edge. The European Slope Current flows eastward along this edge, bringing North Atlantic water into the Norwegian Sea. Some of this water flows south past Shetland into the North Sea. Imagery for July 2021 (Fig. 2c, d) seems to show the bloom is of the latter kind, originating along the northern edge of the shelf.
To confirm the bloom's origins and to understand why 2021 was unusual, we used the OceanParcels software package (Delandmeter and van Sebille, 2019) to simulate the trajectory of virtual particles in the ocean. Particles were initially positioned at 1 m depth along the slope current (Fig. 3). These locations were chosen based on where the speed of the current (from a reanalysis mean climatology for 1993 to 2021) exceeded 0.2 m s−1. OceanParcels modelled 3D movement of the particles, advected by daily mean currents from the NWS reanalysis (product ref. no. 1, Table 1) and using a 3 h time step. This was done separately for each year from 1998–2022, starting with particles in initial positions on 3 April and running forward 3 months to predict positions on 3 July (Fig. 3).
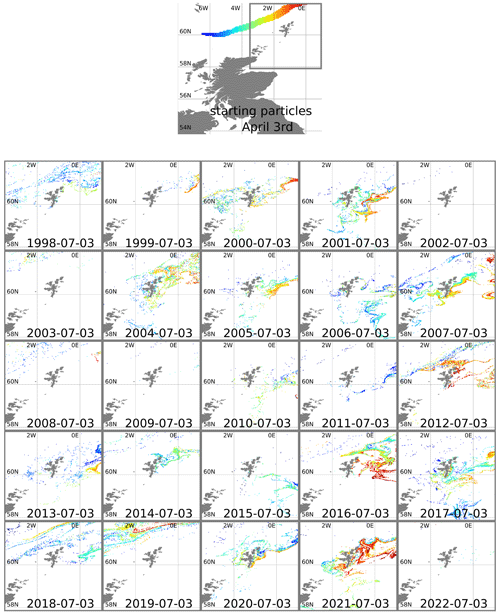
Figure 3Top plot shows 3 April starting positions for particles positioned along the European Slope Current. The area around Shetland is marked with a grey line. Other plots show particle positions in this area advected forward 3 months, using reanalysis surface currents for each year from 1998–2022. Particles are coloured according to longitude of starting position.
In some years nearly all the particles move off beyond the edge of the plots (1999, 2002, 2003, 2009, 2022). In most years all the red particles (those initially at the eastern end of the slope current) disappear in this way. In 2021 it is these red particles that end up close to the eastern side of Shetland on 3 July. The year 2021 was unusual, although not unique, in that easterly winds during spring drove surface currents that pushed particles westward for part of that time. Figure 4 shows 10 m winds (from ERA5) and surface currents (from NWS reanalysis) for May 2021 and for a May climatology. To the east and north of Shetland, climatological winds are westerly, and the surface current is westerly or northerly. For May 2021, winds are north-easterly. These winds induce easterly surface currents (Ekman transport effect).
The years 2012, 2016, and 2019 also experienced easterly winds in spring or early summer (based on ERA5 reanalysis) and similarly show large numbers of red particles still within the plot region on 3 July. Other years see large numbers of other-coloured particles come close inshore, in particular 2007, 2017, and 2020. In the satellite imagery (2017–2020) and Kondrik catalogue (1998–2016), none of these years show coccolithophore blooms that reach into the bays and inlets of eastern Shetland. Imagery for 2020 (not shown) comes the closest, with a bloom 10–20 km away from the coast. The 2020 particle tracking has orange particles in this region, originating from further west on the shelf edge.
We conclude that in some years blooms around Shetland form in water coming from the shelf edge. We cannot conclude that bloom development is always linked to specific locations and timings of source water along the shelf edge.
To understand how the bloom appeared so close inshore, we examined the daily particle trajectories for 2021 (not shown). Particles move south down the eastern side of Shetland during the second half of June. In late June and early July there is a brief period of easterly winds, and the particles are driven in towards the coast. Brief easterlies are not unusual, but these coincided with coccolithophore-laden water near the coast. We suggest that coincidences of timing and weather in 2021 created the unusual phenomenon of a visible coccolithophore bloom on the eastern Shetland coast.
Analysis of the 2021 bloom in the Clyde Sea is hampered by a paucity of observations. Weather stations provide data on the atmospheric conditions. Satellite instruments provide estimates of SST and of chlorophyll, with caveats discussed below. We have found no in situ measurements of conditions within the Clyde Sea itself. Biogeochemical reanalysis data are available (Kay et al., 2021), but their ability to accurately simulate the Clyde Sea is hampered by a lack of data on river discharge. Freshwater input and nutrient input from rivers are important variables for the biogeochemistry here. In this paper we analyse the data that are available and build from them a plausible storyline. That storyline starts with diatom growth in early spring.
4.1 The Clyde Sea and the annual cycle of diatoms
The Clyde Sea comprises a large tidal estuary with several islands and fjord-like sea lochs. It is the outlet of the River Clyde and other rivers into the Irish Sea. It has a maximum depth of 164 m, with a sill (the “Great Plateau”) of approximately 40 m depth where it meets the Irish Sea. Freshwater outflow from rivers and from land drainage tends to maintain stable stratification in the basin (Simpson and Rippeth, 1993). This together with the sill restricts tidal mixing to mostly near-surface waters. Edwards et al. (1986) estimate a residence time of 2 months for surface water in the main body of the Clyde Sea. Nutrient content in the Clyde Sea tends to be higher than adjacent coastal waters (based on measurements of nitrate in Slesser and Turrell, 2013). Tidal currents within the Clyde Sea tend to be weak. Water in the deeper waters below the sill can stagnate, leading to nutrient build-up near the sea bed. Simpson and Rippeth (1993) show that strong winds can sometimes overcome the vertical stability and mix the water column. This would act to replenish nutrients in the surface layers in the event of an algal bloom.
Marshall and Orr (1927) sampled the Clyde Sea and its lochs extensively, finding the following:
There is a well-marked spring diatom maximum which starts at the end of March or the beginning of April.
A diatom bloom will consume nutrients (Stief et al., 2022), which will tend to inhibit further phytoplankton growth (Elser et al., 2007). Marshall and Orr (1927) also observed a second, smaller summer maximum but noted that diatoms near the surface were less healthy than those several metres deeper. Tests with samples left in direct sunlight and in shade showed that summer light levels were injurious for these diatoms.
Hannah and Boney (1983) assessed extensive and more recent surveys (1976–1978) of the Inner Firth. They found rapid growth in diatoms from late March or early April in each year of the study, dominated by Skeletonema spp. and Thalassiosira nordenskioldii. They also found Nitzschia seriata (now called Pseudo-nitzschia “seriata type”) and Chaetoceros spp. at those times present in considerable numbers. During these spring blooms total chlorophyll was dominated by diatoms. For 1977 they found evidence that the Skeletonema were being grazed by microzooplankton (Ebria). Bresnan et al. (2016) also report an intense spring bloom dominated by Skeletonema during the monitoring period 2005–2013.
4.2 Timing and source of 2021 Clyde coccolithophore bloom
Figure 1 shows the Clyde bloom on 21 June 2021. There are earlier visual reports of bright patches in the sea around the Isle of Arran in the centre of the Clyde Sea on 12 June (Evers-King et al., 2021). Satellite imagery (Fig. 2a) has no bright patch in the Clyde Sea that day, but the sea around Arran is partly obscured by cloud. Imagery for 13–16 June is almost wholly obscured by cloud, and the first clear satellite image of a bright patch across the whole of the Clyde Sea is from 18 June (Fig. 2b). This patch persists until 5 July and then fades. Figure 2c shows that by this time the bloom is apparent even in the northernmost reaches of Loch Fyne. This sea loch flows into the Clyde Sea but is tidal along its 65 km length.
Transmission electron microscopy of a sample from Millport collected at the end of June revealed the bloom to be comprised of liths and cells of E. huxleyi – morphotype B (Fig. 1d), providing the first confirmation of this species in high abundance at this site. E. huxleyi morphotypes A and B have been recorded in the waters around Scotland (van Bleijswijk et al., 1991; León et al., 2018). Little is known about the seasonality of E. huxleyi morphotypes on the west coast of Scotland. A study at the Marine Scotland Scottish Coastal Observatory (SCObs) monitoring site at Stonehaven on the east coast from 2010–2013 showed a distinct repeated seasonality in the occurrence of different E. huxleyi morphotypes (León et al., 2018). Morphotype B was commonly recorded in spring, with morphotype A occurring from June to August followed by an overcalcified form of morphotype A (type AO) in autumn and winter months. The dominance of E. huxleyi morphotype B in the 2021 Clyde bloom differs in timing from the seasonality recorded on the east coast.
Coccolithophores have a haplodiplontic life cycle (Keuter et al., 2021). New cells are haploid (one set of chromosomes in the nucleus). These haploid cells develop into diploid cells (two sets of chromosomes in the nucleus). For the genus Emiliania, it is only the diploid form that produces coccoliths. Frada et al. (2008) explain its “Cheshire Cat” strategy for resisting viral attack. Giant phycodnaviruses (Emiliania huxleyi viruses, EhVs) infect and lyse diploid-phase cells and are heavily implicated in the termination of blooms. The diploid cells transition to haploid cells that are resistant to EhVs, shedding coccoliths as they do. Thus the bloom in the Clyde Sea may have started some time before sufficient coccoliths had accumulated to make it visible.
It is possible that E. huxleyi was introduced by tidal mixing into the Clyde Sea from the Irish Sea or that it was already resident. Reverse particle tracking (not shown) excludes immediate seeding from blooms at the Malin shelf break as a likely cause. The satellite imagery shows the bloom mostly confined to the Clyde Sea. We conclude that conditions within the Clyde basin in late May or early June were particularly favourable for E. huxleyi to thrive. We aim here to understand exactly which aspects were favourable and what brought them about.
4.3 Physical environment in 2021
Figure 5a shows daily values of sea surface temperature (SST) from the NWS reanalysis averaged over the Clyde Sea for the years 1998–2021. June 2021 values are in the middle of the range. Values for April and May are towards the cold end of the range. Statistics from a high-resolution satellite SST product in Table 2 (product ref. no. 3, Table 1) confirm this. Monthly means for April, May, and June 2021 are, respectively, at the 10th, 5th, and 52nd percentiles for those months over the period 1982–2021.
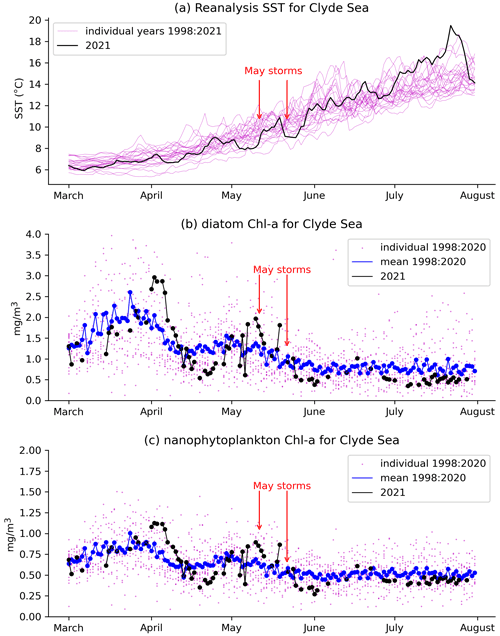
Figure 5 Daily mean values averaged over the Clyde Sea basin of (a) reanalysis sea surface temperature for individual years. The year 2021 is in black. Chlorophyll concentrations (product ref. no. 2, Table 1) for the Clyde Sea from ocean colour products for (b) diatoms and (c) nanophytoplankton are also given. Black dots and lines are ocean colour estimates for 2021. Blue shows mean estimates for 1998 to 2020. Smaller purple dots are values for individual years, showing the year-to-year spread.
Table 2a has monthly statistics of SST from a satellite SST product (product ref. no. 3, Table 1) for a point within the Clyde Sea (55.27∘ N, 5.11∘ W), close to the lower right intersection of grid lines in Fig. 1. April and May 2021 SSTs were unusually cold compared to climatology. June was close to the median. The cold SST can be linked to the weather (Table 2b, c, d). April saw anticyclonic weather that was cold, dry, and exceptionally sunny (Weather Magazine, 2021a). May 2021 had anomalously low atmospheric pressure over the UK, bringing storms, high rainfall, and high winds (Weather Magazine, 2021b). June was drier and warmer than average.
There were two severe storms in May 2021, one on 9, 10, and 11 May and a stronger one on 20 and 21 May. These appear to coincide with periods when there is a pause in the rate of increase in SST (Fig. 5a). This suggests the strong winds are mixing the water column.
Table 2Statistics of monthly means from (a) CMEMS European area level 4 SST analysis at 55.27∘ N, 5.11∘ W, and (b, c, d) from weather stations in the Clyde catchment area (National Climate Information Centre, NCIC).
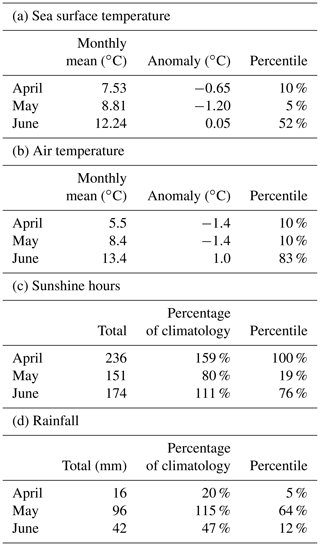
Anomalies and percentiles are relative to yearly climatology: (a) SST is CMEMS reprocessed level 4 satellite product 1982–2020 (product ref. no. 3, Table 1). (b, c, d) Weather station data are for the Clyde catchment area from NCIC for 1980–2021.
4.4 Ocean colour estimates of chlorophyll
Figure 5 shows a time series of estimates of chlorophyll a from the CMEMS ocean colour product (product ref. no. 2, Table 1) for two plankton functional types, diatoms and nanophytoplankton. Mean values for 1998–2020 (blue line) show similar patterns for diatoms and nanophytoplankton: concentrations rising to a peak in late March and a smaller second peak in early May, although with considerable year-to-year variation (purple dots). Estimates for 2021 are again similar between both functional types. Both have strong peaks in early April, well above the 1998–2020 mean. Values drop rapidly during April, rising again towards the end of that month. Both types also show a fall immediately following the two May storms, around 10 and 20 May.
As discussed in Sect. 2.1, chlorophyll estimation can be difficult in coastal waters. Vertical mixing and river discharge due to the May storms might increase levels of dissolved organic matter and sediment in the water. Vertical mixing might dilute plankton in the surface layers that are sensed by the ocean colour instruments. The presence of coccoliths and cloud mean that much of the data for June have been masked in the estimation process. The ocean colour product includes an estimate of root mean square error (RMSE) following Brewin et al. (2017). For the Clyde Sea, values of RMSE for diatoms and nanophytoplankton in both April and May 2021 are given as approximately 0.5 mg m−3. This is similar in size to the estimates themselves for nanophytoplankton, and so we avoid drawing conclusions from Fig. 5c. Estimated concentrations for diatoms are somewhat larger, and so we have more confidence in drawing conclusions from the diatom time series (Fig. 5b).
4.5 Possible causes of Clyde bloom
Mayers et al. (2019) assessed coccolithophore growth and mortality rates based on samples from the Celtic Sea in April 2015. They identified several conditions that favour coccolithophore blooms. These are considered individually below.
- (a) Warm, stratified waters.
-
SST in June 2021 was close to average for that month (Table 2a). Vertical profiles of temperature from the reanalysis for 2021 (not shown) are stably stratified, but this is typical for June. The following conclusion was reached: June 2021 temperatures were not unusual.
- (b) Sunlight.
-
June 2021 was sunnier than average (76th percentile, Table 2c). The following conclusion was reached: sunshine might have been a contributory factor in 2021.
- (c) Availability of nutrients.
-
We have no direct measurements of nutrients in the Clyde Sea. April 2021 was a dry month (5th percentile, Table 2d), and so river discharge in April would have been low. Rainfall in May was above average. May was also a stormy month, which may have mixed the water column, bringing nutrients from deep water into the photic zone (Pingree et al., 1977). Each of these factors could lead to higher-than-usual nutrient availability by the end of May. Ocean colour estimates of diatoms suggest lower-than-usual diatom growth in April and increased growth during May (Fig. 5b). The following conclusion was reached: observations show low chlorophyll mass in spring 2021. This could be due to the cold water temperatures and limited nutrient input in April 2021. The wet and stormy conditions in May of both years likely increased nutrient levels in near-surface layers in the Clyde Sea. Both these factors would help produce suitable conditions for a bloom.
- (d) Scarcity of predatory microzooplankton.
-
Mayers et al. (2019) found that microzooplankton exert strong top-down control on coccolithophore populations, grazing up to 80 % of daily production in a bloom of E. huxleyi. We might hypothesise that fewer diatoms in April and May led to low numbers of microzooplankton during that time, reducing the grazing pressure on E. huxleyi in late May and early June. However, growth rates for microzooplankton can be rapid, sometimes more than three doublings per day for tintinnids (Verity, 1986). The following conclusion was reached: this could be a contributory factor, though we have no evidence for this. The ability of microzooplankton to multiply rapidly suggests at least that other factors were also involved.
Advection of a bloom into the region is another possible cause. We consider this unlikely for two reasons. The Clyde Sea is semi-enclosed, with an estimated residence time of 2 months for surface water in the main body of the Clyde Sea (Edwards et al., 1986). This was consistent with reverse tracking (not shown) of a set of virtual particles placed in the Clyde Sea in June and tracked backwards for 60 d to find their source. The majority remained within the Clyde Sea. Also, satellite imagery shows a bloom in the Clyde Sea but not in the adjoining Irish Sea.
Phytoplankton are of special interest in the waters around Scotland, where aquaculture and fishing are major industries. Through primary production, phytoplankton form the base of a food chain that sustains marine fauna (Frederiksen et al., 2006). Blooms of phytoplankton can be harmful to other marine life and can produce toxins dangerous for human consumers of seafood (Davidson et al., 2011). There is thus increasing interest from policy-makers to understand the diversity dynamics of phytoplankton communities in Scotland and other parts of the North West Shelf (NWS) and to understand its influence on industries and diversity status assessments (Siemering et al., 2016; McQuatters-Gollop et al., 2019).
This paper presents hypotheses to explain two unusual blooms. We suggest that the bloom on the eastern side of Shetland originated in Atlantic water brought north of Shetland by the European Slope Current. The water's passage eastward was retarded by a period of anomalous easterly winds in May, and it was later steered towards the Shetland coast by a shorter period of easterly winds. The timing was such that there were abundant coccoliths present when this water was close inshore. The bloom within the Clyde Sea appears to have developed in place. We hypothesise that environmental factors may have combined to create suitable conditions in the Clyde Sea. A cold and dry April could have restricted spring growth of diatoms, leaving nutrients available for a summer bloom of coccolithophores. A wet and stormy May might also have added to the nutrients.
Our explanations are based on limited evidence (SST and chlorophyll estimates from satellites, modelling by the reanalysis). We do not have in situ measurements from within the blooms to confirm our hypotheses.
For both blooms, we propose the weather as a key factor. Other studies also identify the importance of the weather for algal blooms. Whyte et al. (2014) looked at unusually strong blooms of the biotoxin-producing dinoflagellate Dinophysis on the western side of the Shetland Islands in the summers of 2006 and 2013. They found these blooms coincided with periods where the winds, usually more southerly, became westerly. They suggested the westerly winds advected Dinophysis populations onshore, resulting in an increase in diarrhetic shellfish toxin levels in farmed mussels (Mytilus edulis).
There is evidence that the distribution of coccolithophores has expanded polewards in recent decades (Beaugrand et al., 2013; Winter et al., 2013; Rivero-Calle et al., 2015), due either to changes in ocean temperature or dissolved inorganic carbon. Growth of E. huxleyi is also known to be impacted by major changes in ocean pH (Riebesell et al., 2017). Changes in pH observed in seas around the UK (Findlay et al., 2022) are not large enough to explain recent variability in coccolithophore abundance in Scottish waters.
Changing weather patterns have the potential to influence the occurrence of unusual phytoplankton blooms in coastal waters. These changes have the potential to impact higher trophic levels in the marine ecosystem. A better understanding of the processes and dynamics involved will help in forewarning, preparation, and development of adaptation measures for these changes.
This paper shows how use of satellite data and model reanalysis can help to meet the challenge of assessing major events in UK waters, despite a sparsity of in situ observations. However we could be more confident in our findings if we had more information about environmental conditions. More complete data on river discharge would help in simulating biogeochemical and ecosystem variables in the Clyde Sea and other inshore water bodies. Widespread routine monitoring of nutrient levels and phytoplankton components could help greatly in understanding future blooms.
The data products used in this article, as well as their names, availability, and documentation, are summarised in Table 1.
RR processed the reanalysis data and wrote much of the text. EB produced the micrograph of the E. huxleyi sample. PM produced the ocean colour images. Every author contributed to discussion and development of the hypotheses presented. Every author also added to and reviewed the text.
The contact author has declared that none of the authors has any competing interests.
Publisher’s note: Copernicus Publications remains neutral with regard to jurisdictional claims in published maps and institutional affiliations.
We gratefully acknowledge use of the OceanParcels code (Delandmeter and van Sebille, 2019) in calculating backward trajectories. We also acknowledge use of collated station statistics from the UK National Climate Information Centre and ERA5 reanalysis data (Hersbach et al., 2023) downloaded from the Copernicus Climate Change Service (C3S) Climate Data Store. We thank ESA for the satellite image in Fig. 1, made available under Creative Commons License BY-SA 3.0 IGO (https://creativecommons.org/licenses/by-sa/3.0/igo/, last access: 30 May 2023). Transmission electron analysis of the Millport water sample was performed at the Microscopy Unit, Institute of Medical Sciences, University of Aberdeen.
This paper was edited by Griet Neukermans and reviewed by David Bowers and two anonymous referees.
Autret, E., Tandéo, P., Paul, F., and Piollé, J. F.: EU Copernicus Marine Service Quality Information Document for the European North West Shelf/Iberia Biscay Irish Seas – High Resolution L4 Sea Surface Temperature Reprocessed, SST_ATL_SST_L4_REP_OBSERVATONS_010_026, Issue 1.4, Mercator Ocean International, https://catalogue.marine.copernicus.eu/documents/QUID/CMEMS-SST-QUID-010-026.pdf, last access: 12 April 2023, 2021a. a
Autret, E., Piollé, J. F., Tandéo, P., and Prévost, C.: EU Copernicus Marine Service Product User Manual for the European North West Shelf/Iberia Biscay Irish Seas – High Resolution L4 Sea Surface Temperature Reprocessed, SST_ATL_SST_L4_REP_OBSERVATONS_010_026, Issue 1.4, Mercator Ocean International, https://catalogue.marine.copernicus.eu/documents/PUM/CMEMS-SST-PUM-010-026.pdf (last access: 12 April 2023), 2021b. a
Balch, W. M., Bowler, B. C., Drapeau, D. T., Lubelczyk, L. C., Lyczkowski, E., Mitchell, C., and Wyeth, A.: Coccolithophore distributions of the North and South Atlantic Ocean, Deep Sea Research Part I: Oceanographic Research Papers, 151, 103 066, https://doi.org/10.1016/j.dsr.2019.06.012, 2019. a
Beaugrand, G., McQuatters-Gollop, A., Edwards, M., and Goberville, E.: Long-term responses of North Atlantic calcifying plankton to climate change, Nature Climate Change, 3, 263–267, https://doi.org/10.1038/nclimate1753, 2013. a
Bowers, D., Harker, G., Smith, P., and Tett, P.: Optical Properties of a Region of Freshwater Influence (The Clyde Sea), Estuarine, Coastal and Shelf Science, 50, 717–726, https://doi.org/10.1006/ecss.1999.0600, 2000. a
Bradshaw, H.: Marine algae turned Scotland's water bright blue, BBC News Online, https://www.bbc.co.uk/news/uk-scotland-57720790 (last access: 30 May 2023), 2021. a
Bresnan, E., Cook, K., Hindson, J., Hughes, S., Lacaze, J. P., Walsham, P., Webster, L., and Turrell, W. R.: The Scottish coastal observatory 1997-2013 part 2: description of Scotland's coastal waters, Scottish Marine and Freshwater Science, 7, 278, https://doi.org/10.7489/1881-1, 2016. a
Brewin, R. J. W., Ciavatta, S., Sathyendranath, S., Jackson, T., Tilstone, G., Curran, K., Airs, R. L., Cummings, D., Brotas, V., Organelli, E., Dall'Olmo, G., and Raitsos, D. E.: Uncertainty in Ocean-Color Estimates of Chlorophyll for Phytoplankton Groups, Front. Mar. Sci., 4, 104, https://doi.org/10.3389/fmars.2017.00104, 2017. a
Collela, S., Böhm, E., Cesarini, C., Garnesson, P., Netting, J., and Calton, B.: EU Copernicus Marine Service Product User Manual for North Atlantic Ocean Colour Plankton, Reflectance, Transparency and Optics MY L3 daily observations, Issue 3.0, Mercator Ocean International, https://catalogue.marine.copernicus.eu/documents/PUM/CMEMS-OC-PUM.pdf (last access: 12 April 2023), 2022. a
Copernicus Climate Change Service, Climate Data Store: ERA5 hourly data on single levels from 1940 to present, Copernicus Climate Change Service (C3S) Climate Data Store (CDS) [data set], https://doi.org/10.24381/cds.adbb2d47, 2023. a
Davidson, K., Tett, P., and Gowen, R.: Chapter 4 Harmful Algal Blooms, in: Marine Pollution and Human Health, pp. 95–127, The Royal Society of Chemistry, https://doi.org/10.1039/9781849732871-00095, 2011. a
Delandmeter, P. and van Sebille, E.: The Parcels v2.0 Lagrangian framework: new field interpolation schemes, Geosci. Model Dev., 12, 3571–3584, https://doi.org/10.5194/gmd-12-3571-2019, 2019. a, b
Edwards, A., Baxter, M. S., Ellett, D. J., Martin, J. H. A., Meldrum, D. T., and Griffiths, C. R.: Clyde Sea hydrography, Proc. Roy. Soc. Edinburgh. B, 90, 67–83, https://doi.org/10.1017/S0269727000004887, 1986. a, b
Elser, J. J., Bracken, M. E., Cleland, E. E., Gruner, D. S., Harpole, W. S., Hillebrand, H., Ngai, J. T., Seabloom, E. W., Shurin, J. B., and Smith, J. E.: Global analysis of nitrogen and phosphorus limitation of primary producers in freshwater, marine and terrestrial ecosystems, Ecol. Lett., 10, 1135–1142, https://doi.org/10.1111/j.1461-0248.2007.01113.x, 2007. a
EU Copernicus Service Product: Atlantic – European North West Shelf – Ocean Physics Reanalysis, Mercator Ocean International, Copernicus Services [data set], https://doi.org/10.48670/moi-00059, 2021a. a
EU Copernicus Service Product: European North West Shelf/Iberia Biscay Irish Seas – High Resolution L4 Sea Surface Temperature Reprocessed, Mercator Ocean International, Copernicus Services [data set], https://doi.org/10.48670/moi-00153, 2021b. a
EU Copernicus Service Product: North Atlantic Ocean Colour Plankton, Reflectance, Transparency and Optics MY L3 daily observations, Mercator Ocean International, Copernicus Services [data set], https://doi.org/10.48670/moi-00286, 2022. a
Evers-King, H., Miller, P., Loveday, B., and Wallace, L.: Ocean colour data reveals the extent of beautiful blue waters associated with a phytoplankton bloom observed by hikers around the Isle of Arran in June 2021, EUMETSAT press release, August 2021, https://www.eumetsat.int/big-bloom-firth-clyde (last access: 30 May 2023), 2021. a
Findlay, H., Artoli, Y., Birchenough, S., Hartman, S., León, P., and Stiasny, M.: Climate change impacts on ocean acidification relevant to the UK and Ireland, MCCIP Sci. Rev., 2022, 24 pp., https://doi.org/10.14465/2022.reu03.oac, 2022. a
Frada, M., Probert, I., Allen, M. J., Wilson, W. H., and de Vargas, C.: The “Cheshire Cat” escape strategy of the coccolithophore Emiliania huxleyi in response to viral infection, P. Natl. Acad. Sci. USA, 105, 15944–15949, https://doi.org/10.1073/pnas.0807707105, 2008. a, b
Frederiksen, M., Edwards, M., Richardson, A. J., Halliday, N. C., and Wanless, S.: From plankton to top predators: bottom-up control of a marine food web across four trophic levels, J. An. Ecol., 75, 1259–1268, https://doi.org/10.1111/j.1365-2656.2006.01148.x, 2006. a
Garnesson, P., Mangin, A., Bretagnon, M., and Jutard, Q.: EU Copernicus Marine Service Quality Information Document for the North Atlantic Ocean Colour Plankton, Reflectance, Transparency and Optics MY L3 daily observations, OCEANCOLOUR_ATL_BGC_L3_MY_009_113, Issue 3.0, Mercator Ocean International, https://catalogue.marine.copernicus.eu/documents/QUID/CMEMS-OC-QUID-009-111to114-121to124.pdf (last access: 12 April 2023), 2022. a
Hannah, F. and Boney, A.: Nanophytoplankton in the Firth of Clyde, Scotland: Seasonal abundance, carbon fixation and species composition, J. Exp. Mar. Biol. Ecol., 67, 105–147, https://doi.org/10.1016/0022-0981(83)90085-0, 1983. a
Hersbach, H., Bell, B., Berrisford, P., Biavati, G., Horányi, A., Muñoz-Sabater, J., Nicolas, J., Peubey, C., Radu, R., Rozum, I., Schepers, D., Simmons, A., Soci, C., Dee, D., and Thépaut, J.-N.: ERA5 hourly data on single levels from 1940 to present, Copernicus Climate Change Service (C3S) Climate Data Store (CDS), https://doi.org/10.24381/cds.adbb2d47, 2023. a, b, c
Highfield, A., Evans, C., Walne, A., Miller, P. I., and Schroeder, D. C.: How many Coccolithovirus genotypes does it take to terminate an Emiliania huxleyi bloom?, Virology, 466–467, 138–145, https://doi.org/10.1016/j.virol.2014.07.017, special issue: Giant Viruses, 2014. a
Johns, C., Bondoc-Naumovitz, K., Matthews, A., Matson, P., Iglesias-Rodriguez, M. D., Taylor, A., Fuchs, H., and Bidle, K.: Adsorptive exchange of coccolith biominerals facilitates viral infection, Sci. Adv., 9, eadc8728, https://doi.org/10.1126/sciadv.adc8728, 2023. a
Kay, S., McEwan, R., and Ford, D.: EU Copernicus Marine Service Quality Information Document, North West European Shelf Production Centre NWSHELF_MULTIYEAR_BIO_004_011, Issue 5.1, Mercator Ocean International, https://catalogue.marine.copernicus.eu/documents/QUID/CMEMS-NWS-QUID-004-011.pdf (last access: 12 April 2023), 2021. a
Keuter, S., Young, J. R., Koplovitz, G., Zingone, A., and Frada, M. J.: Novel heterococcolithophores, holococcolithophores and life cycle combinations from the families Syracosphaeraceae and Papposphaeraceae and the genus Florisphaera, J. Micropalaeontol., 40, 75–99, https://doi.org/10.5194/jm-40-75-2021, 2021. a
Kondrik, D., Kazakov, E., and Pozdnyakov, D.: A synthetic satellite dataset of the spatio-temporal distributions of Emiliania huxleyi blooms and their impacts on Arctic and sub-Arctic marine environments (1998–2016), Earth Syst. Sci. Data, 11, 119–128, https://doi.org/10.5194/essd-11-119-2019, 2019. a, b
León, P., ad Eileen Bresnan, P. W., Hartman, S., Hughes, S., Mackenzie, K., and Webster, L.: Seasonal variability of the carbonate system and coccolithophore Emiliania huxleyi at a Scottish Coastal Observatory monitoring site, Estuarine, Coast. Shelf Sci., 202, 302–314, https://doi.org/10.1016/j.ecss.2018.01.011, 2018. a, b
Madec, G. and Team, N. S.: NEMO ocean engine, Zenodo, https://doi.org/10.5281/zenodo.1464816, 2008. a
Marsh, R., Haigh, I. D., Cunningham, S. A., Inall, M. E., Porter, M., and Moat, B. I.: Large-scale forcing of the European Slope Current and associated inflows to the North Sea, Ocean Sci., 13, 315–335, https://doi.org/10.5194/os-13-315-2017, 2017. a
Marshall, S. M. and Orr, A. P.: The Relation of the Plankton to some Chemical and Physical Factors in the Clyde Sea Area, J. Mar. Biol. Assoc. UK, 14, 837–868, https://doi.org/10.1017/S0025315400051110, 1927. a, b
Mayers, K., Poulton, A., Daniels, C., Wells, S., Woodward, E., Tarran, G., Widdicombe, C., Mayor, D., Atkinson, A., and Giering, S.: Growth and mortality of coccolithophores during spring in a temperate Shelf Sea (Celtic Sea, April 2015), Prog. Oceanogr., 177, 101928, https://doi.org/10.1016/j.pocean.2018.02.024, shelf Sea Biogeochemistry: Pelagic Processes., 2019. a, b
McQuatters-Gollop, A., Atkinson, A., Aubert, A., Bedford, J., Best, M., Bresnan, E., Cook, K., Devlin, M., Gowen, R., Johns, D. G., Machairopoulou, M., McKinney, A., Mellor, A., Ostle, C., Scherer, C., and Tett, P.: Plankton lifeforms as a biodiversity indicator for regional-scale assessment of pelagic habitats for policy, Ecol. Indic., 101, 913–925, https://doi.org/10.1016/j.ecolind.2019.02.010, 2019. a
Mogensen, K., Balmaseda, M. A., and Weaver, A.: The NEMOVAR ocean data assimilation system as implemented in the ECMWF ocean analysis for System 4, ECMWF Technical Memoranda, https://doi.org/10.21957/x5y9yrtm, 2012. a
Monteiro, F. M., Bach, L. T., Brownlee, C., Bown, P., Rickaby, R. E. M., Poulton, A. J., Tyrrell, T., Beaufort, L., Dutkiewicz, S., Gibbs, S., Gutowska, M. A., Lee, R., Riebesell, U., Young, J., and Ridgwell, A.: Why marine phytoplankton calcify, Sci. Adv., 2, e1501822, https://doi.org/10.1126/sciadv.1501822, 2016. a
Müller, M. N.: On the Genesis and Function of Coccolithophore Calcification, Front. Mar. Sci., 6, https://doi.org/10.3389/fmars.2019.00049, 2019. a
OC-CCI: Product User Guide v5.0, Ocean Colour Climate Change Initiative, ESA, D4.2, https://doi.org/10.5285/1dbe7a109c0244aaad713e078fd3059a, 2020. a
Pingree, R. D., Maddock, L., and Butler, E. I.: The influence of biological activity and physical stability in determining the chemical distributions of inorganic phosphate, silicate and nitrate, J. Mar. Biolo. Assoc. UK, 57, 1065–1073, https://doi.org/10.1017/S0025315400026138, 1977. a
Renshaw, R., Wakelin, S., Golbeck, I., and O'Dea, E.: EU Copernicus Marine Service Quality Information Document for the Atlantic – European North West Shelf – Ocean Physics Reanalysis, NWSHELF_MULTIYEAR_PHY_004_009, Issue 5.2, Mercator Ocean International, https://catalogue.marine.copernicus.eu/documents/QUID/CMEMS-NWS-QUID-004-009.pdf (last access: 12 April 2023), 2021. a, b, c
Riebesell, U., Bach, L. T., Bellerby, R. G. J., Monsalve, J. R. B., Boxhammer, T., Czerny, J., Larsen, A., Ludwig, A., and Schulz, K. G.: Competitive fitness of a predominant pelagic calcifier impaired by ocean acidification, Nat. Geosci., 10, 19–23, https://doi.org/10.1038/ngeo2854, 2017. a
Rivero-Calle, S., Gnanadesikan, A., Castillo, C. E. D., Balch, W. M., and Guikema, S. D.: Multidecadal increase in North Atlantic coccolithophores and the potential role of rising CO2, Science, 350, 1533–1537, https://doi.org/10.1126/science.aaa8026, 2015. a
Röhrs, J., Sutherland, G., Jeans, G., Bedington, M., Sperrevik, A. K., Dagestad, K.-F., Gusdal, Y., Mauritzen, C., Dale, A., and LaCasce, J. H.: Surface currents in operational oceanography: Key applications, mechanisms, and methods, J. Oper. Oceanogr., 16, 60–88, https://doi.org/10.1080/1755876X.2021.1903221, 2023. a
Rost, B. and Riebesell, U.: Coccolithophores and the biological pump: responses to environmental changes, 99–125, Springer Berlin Heidelberg, Berlin, Heidelberg, https://doi.org/10.1007/978-3-662-06278-4_5, 2004. a
Siemering, B., Bresnan, E., Painter, S. C., Daniels, C. J., Inall, M., and Davidson, K.: Phytoplankton Distribution in Relation to Environmental Drivers on the North West European Shelf Sea, PLOS ONE, 11, 1–24, https://doi.org/10.1371/journal.pone.0164482, 2016. a
Simpson, J. and Rippeth, T.: The Clyde Sea: a Model of the Seasonal Cycle of Stratification and Mixing, Estuarine, Coast. Shelf Sci., 37, 129–144, https://doi.org/10.1006/ecss.1993.1047, 1993. a, b
Slesser, G. and Turrell, W.: Annual Cycles of Physical, Chemical and Biological Parameters in Scottish Waters, Scottish Marine and Freshwater Science, 4, 17 pp., https://doi.org/10.7489/1511-1, 2013. a
Stief, P., Schauberger, C., Lund, M. B., Greve, A., Abed, R. M. M., Al-Najjar, M. A. A., Attard, K., Bonaglia, S., Deutzmann, J. S., Franco-Cisterna, B., García-Robledo, E., Holtappels, M., John, U., Maciute, A., Magee, M. J., Pors, R., Santl-Temkiv, T., Scherwass, A., Sevilgen, D. S., de Beer, D., Glud, R. N., Schramm, A., and Kamp, A.: Intracellular nitrate storage by diatoms can be an important nitrogen pool in freshwater and marine ecosystems, Commun. Earth Environ., 3, 154–164, https://doi.org/10.1038/s43247-022-00485-8, 2022. a
Tonani, M., Ascione, I., and Saulter, A.: EU Copernicus Marine Service Product User Manual for the North West European Shelf Ocean Physical and Biogeochemical Reanalysis NWSHELF_MULTIYEAR_PHY_004_009, NWSHELF_MULTIYEAR_BGC_004_011, Issue 1.3, Mercator Ocean International, https://catalogue.marine.copernicus.eu/documents/PUM/CMEMS-NWS-PUM-004-009-011.pdf (last access: 12 April 2023), 2022. a
van Bleijswijk, J., van der Wal, P., Kempers, R., Veldhuis, M., Young, J. R., Muyzer, G., de Vrind-de Jong, E., and Westbroek, P.: Distribution of two types of Emiliania Huxleyi (Prymnesiophyceae) in the North East Atlantic region as determined by Immunofluorescence and Coccolith Morphology 1, J. Phycology, 27, 566–570, https://doi.org/10.1111/j.0022-3646.1991.00566.x, 1991. a
Verity, P. G.: Growth rates of natural tintinnid populations in Narragansett Bay, Mar. Ecol. Prog. Series, 29, 117–126, https://doi.org/10.3354/meps029117, 1986. a
Voss, K. J., Balch, W. M., and Kilpatrick, K. A.: Scattering and attenuation properties of Emiliania huxleyi cells and their detached coccoliths, Limnol. Oceanogr., 43, 870–876, https://doi.org/10.4319/lo.1998.43.5.0870, 1998. a
Waters, J., Lea, D. J., Martin, M. J., Mirouze, I., Weaver, A., and While, J.: Implementing a variational data assimilation system in an operational 1/4 degree global ocean model, Q. J. Roy. Meteorol. Soc., 141, 333–349, https://doi.org/10.1002/qj.2388, 2015. a
Weather Magazine 2021a: April 2021 Most places cold, sunny and very dry, Weather, 76, i–iv, https://doi.org/10.1002/wea.4005, 2021. a
Weather Magazine 2021b: May 2021 Cold, dull and wet, Weather, 76, i–iv, https://doi.org/10.1002/wea.4026, 2021. a
Whyte, C., Swan, S., and Davidson, K.: Changing wind patterns linked to unusually high Dinophysis blooms around the Shetland Islands, Scotland, Harmful Algae, 39, 365–373, https://doi.org/10.1016/j.hal.2014.09.006, 2014. a
Winter, A., Henderiks, J., Beaufort, L., Rickaby, R. E. M., and Brown, C. W.: Poleward expansion of the coccolithophore Emiliania huxleyi, J. Plankton Res., 36, 316–325, https://doi.org/10.1093/plankt/fbt110, 2013. a
Young, J. R., Davis, S. A., Bown, P. R., and Mann, S.: Coccolith Ultrastructure and Biomineralisation, J. Struct. Biol., 126, 195–215, https://doi.org/10.1006/jsbi.1999.4132, 1999. a