the Creative Commons Attribution 4.0 License.
the Creative Commons Attribution 4.0 License.
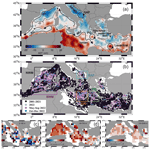
Subsurface warming derived from Argo floats during the 2022 Mediterranean marine heat wave
Riccardo Martellucci
Antonella Gallo
Elisabeth Kubin
Elena Mauri
Mélanie Juza
Giulio Notarstefano
Massimo Pacciaroni
Antonio Bussani
Milena Menna
The Mediterranean marine heat wave (MHW) during the warm season (May–September) and the fall period (October–December) of 2022 is analyzed using Argo float in situ observations in the upper 2000 m of depth. The five study regions (North Western Mediterranean, South Western Mediterranean, central Ionian Sea, Pelops Gyre, and South Adriatic Pit) most affected by warming in different layers were selected and investigated. The primary goal is to provide insights into how the water column responds to the onset and progression of the MHW during the warming period, characterized by peak stratification and reduced vertical mixing. Additionally, this study aims to examine how the heat accumulated in the upper layers is redistributed to deep layers within regions with different dynamic characteristics through advection and/or mixing during the subsequent fall period.
Temperature anomaly profiles, Ta (z), computed for each area and for both periods were divided into three categories based on vertical heat penetration: Category 1 (shallow, 0–150 m), Category 2 (intermediate, 150–700 m), and Category 3 (deep, >700 m). During the warm season, Category 1 profiles had a temperature anomaly near zero or slightly negative in a thin layer between 50 and 150 m depth, while warming was observed in the 0–50 m layer and below the middle layer. Profiles characterized by greater vertical heat penetration (categories 2 and 3) were mainly in mesoscale or subbasin structures and showed the largest positive temperature anomaly in the surface and intermediate layers. All profile categories showed a warming between 200 and 800 m depth. This increase is roughly split, with half attributed to the impact of the 2022 MHW and the other half linked to the ongoing long-term trend in ocean temperatures. During the fall period and in the layer below 200 m depth, the shapes of the Ta profiles are similar for all sectors with the exception of the South Adriatic Pit, which depicts a +0.5 °C warming at 800 m depth.
The present work highlights the warming characteristics throughout the entire water column across different regions of the Mediterranean Sea, and it seeks to connect the impacts of the warm season on the cold period with oceanic dynamic processes, such as dense water formation, upwelling, or water column stratification. These regions are characterized by dynamic activities (e.g., dense water formation, upwelling); therefore, any variation in these ocean processes can influence the thermohaline circulation and, consequently, the climate system.
- Article
(6049 KB) - Full-text XML
- BibTeX
- EndNote
Marine heat waves (MHWs) are extreme ocean temperature events occurring over extended periods of time (Hobday et al., 2016). Over the past decade, the frequency of MHW events has increased by 50 % (IPCC, 2023), as well as their duration and magnitude (Oliver et al., 2018). They can affect small areas of coastline or span multiple ocean areas across latitudes with significant impacts on ecosystems, coastal communities, and economies (Wernberg et al., 2013; Garrabou et al., 2022; Dayan et al., 2023).
Since the beginning of the 21st century, the particularly rapid warming trend of the Mediterranean Sea surface layer has been associated with a strong increase in MHW events (Bensoussan et al., 2019; Ibrahim et al., 2021; Juza et al., 2022; Pastor and Khodayar, 2023; Dayan et al., 2023). Several studies, with measurements mainly confined at the surface, have addressed this topic covering different aspects of MHWs using satellite observations and model simulations. In particular, from basin to subregional scale, previous studies have analyzed MHW drivers and indicators; estimated the frequency, duration, and intensity of MHWs; evaluated their trends; and assessed the risks for and impacts on ecosystems (Darmaraki et al., 2019; Galli et al., 2017; Garrabou et al., 2022; Juza et al., 2022; Dayan et al., 2023; Martínez et al., 2023; Marullo et al., 2023; Pastor and Khodayar, 2023; Simon et al., 2023). However, MHWs are not exclusively limited to the surface layer, but they can also propagate throughout the deeper layers of the water column (Darmaraki et al., 2019; Hu et al., 2021; Scannell et al., 2020; Juza et al., 2022). This can cause negative ecological consequences, compromising the maintenance of biodiversity, the provision of food, and the regulation of air quality (Garrabou et al., 2022; Holbrook et al., 2020; Santora et al., 2020; Smale et al., 2019; Schaeffer and Roughan, 2017; Liquete et al., 2016; Martín-López et al., 2016; Mills et al., 2013). A recent study in the Mediterranean Sea shows that although MHW frequency is higher at the surface, the maximum intensity and duration of MHWs are registered in the subsurface layers (Dayan et al., 2023). Moreover, in situ data collected in the tropical western Pacific Ocean show that the maximum intensity of almost every MHW event is found in the subsurface layer, and many of the MHWs occurred even when no significant warming anomalies were detected at the surface (Hu et al., 2021). Using satellite data, Marullo et al. (2023) defined the occurrence of an event in the Mediterranean Sea from May 2022 to spring 2023, with higher intensity in summer 2022 and in the band from 0 to 25° E. Starting from this result, the present work analyzes the subsurface properties of the 2022 MHW in the upper 2000 m depth using in situ hydrographic Argo profiles (product ref. no. 1, Table 1; Wong et al., 2020), collected during the period of highest intensity (warm season, May–September) and in the period thereafter (cold season, October–December). Focusing on the results by Marullo et al. (2023) and on the availability of Argo float profiles, five study areas were selected for our analysis (Fig. 1b).
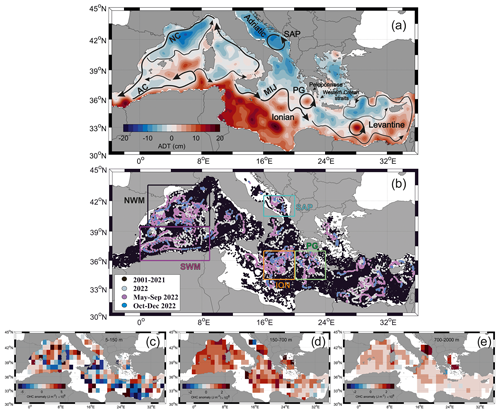
Figure 1(a) Absolute dynamic topography (colors) averaged for the warm season (May–September 2022) along with schematic pathways (black arrows) of the Algerian Current (AC), Northern Current (NC), Mid-Ionian Jet (MIJ), South Adriatic Pit (SAP), and Pelops Gyre (PG); (b) Argo float positions for the whole Mediterranean Sea. Black, magenta, cyan, orange, and green boxes indicate the North West Mediterranean (NWM: 39.5–43.5° N and 1–9° E), South West Mediterranean (SWM: 36–39.5° N and 0–9° E), South Adriatic Pit (SAP: 40.5–42.5° N and 16–20° E), Ionian (ION: 34–37° N and 16–20° E), and Pelops Gyre (PG: 34–37° N and 20–24° E) areas, respectively. (c–e) The 2022 ocean heat content (OHC) anomaly estimated every meter with respect to the 2001–2018 FLOAT climatology period from Argo float profiles in different layers: (c) 5–150, (d) 150–700, and (e) 700–2000 m.
Based on the vertical heat penetration (MHW depth; see the Methods section), the temperature profiles collected in May–September 2022 from each study area were divided into three categories (shallow, intermediate, and deep penetration), and the median profile of temperature anomaly () was computed for each of them. Changes in the vertical temperature anomalies were described and analyzed in relation to the ocean stratification, circulation, and dynamics of each specific area. Lastly, this study examines the properties of the water column during the fall period and speculates on its relationship with the dynamics of the previous warm season's MHW. An estimation of the horizontal and vertical distributions of the ocean heat content (OHC) anomaly in 2022 was also made in the whole Mediterranean Sea (Fig. 1c–e).
The vertical propagation of the 2022 MHW in the Mediterranean Sea was investigated using temperature data collected by Argo floats in the period 2001–2022 (Fig. 1b). These data were collected and made freely available by the international Argo program (which is part of the Global Ocean Observing System; Argo, 2023) and by the national program Argo-Italy that contributes to it (https://argo.ucsd.edu, last access: 23 April 2023; https://www.ocean-ops.org, last access: 23 April 2023).
A comprehensive characterization of the event over the whole Mediterranean Sea was performed starting from the OHC analysis. The OHC, defined as the total amount of heat absorbed and stored by the ocean, can be considered a good indicator for assessing the Earth's energy imbalance (Von Schuckmann et al., 2016). A float-derived OHC climatology (OHC2001–2018) for the period 2001–2018 was estimated in 1° × 1° bins and in different layers (0–150, 150–700, 700–2000 m) using the method by Kubin et al. (2023). Subsequently, Argo temperature data collected in 2022 were averaged on the same grid as OHC2001–2018 to compute the 2022 OHC (OHC2022). The OHCA2022 (ocean heat content anomaly) was then calculated as the difference between the OHC2022 and OHC2001–2018 fields.
The five Mediterranean Sea regions most affected by surface warming (Fig. 1b) were selected using the results of Marullo et al. (2023) and considering the availability of float data. In these regions, we analyzed the vertical penetration of the 2022 MHW signal in the water column during both the warm and cold seasons. The regions selected are the following: the North Western Mediterranean (NWM), the South Western Mediterranean (SWM), the Ionian (ION), the Southern Adriatic Pit (SAP), and the Pelops Gyre (PG) sectors.
The temperature anomaly Ta at each depth z and for each profile and sector was computed as
where T(z) is the 2022 temperature derived from Argo floats, while is the climatological (1985–2018) averaged temperature derived from the SeaDataCloud (SDC) dataset (product ref. no. 4, Table 1; denoted SDC climatology). Specifically, the gridded (0.125° × 0.125°) monthly climatological profiles were linearly interpolated in depth (every 10 m) and at the position of each float profile. Moreover, to compare the 2022 MHW event with the averaged conditions estimated by floats in the selected sectors, Ta profiles were also computed for the whole float dataset in the period 2001–2018 (denoted FLOAT climatology). It is important to highlight that while this study utilizes the SDC climatology, the FLOAT climatology was utilized to facilitate a straightforward comparison with the OHC findings from Kubin et al. (2023). The time window used for the present work (May–September 2022) was chosen based on the latest European Space Agency specification (https://www.esa.int/Applications/Observing_the_Earth/Mediterranean_Sea_hit_by_marine_heatwave, last access: 18 February 2023) and on the estimations by Marullo et al. (2023). These estimations indicate that the 2022 MHW developed in the second half of April in the northwest Mediterranean Sea and extended over the central Mediterranean into September. In this period, Ta profiles were quality-controlled to remove any inconsistency (e.g., profiles with negative surface anomalies) and used to estimate the vertical propagation of the MHW (or MHW depth), following the method by Elzahaby and Schaeffer (2019). For each profile, the positive threshold depth (hereafter ZN) is defined as the depth at which the first negative or zero temperature anomaly occurred:
Knowing ZN, the vertical cumulative temperature anomaly (CTa), defined as
with Δz=10 m, was computed for each profile from the surface (z=0) to the positive threshold depth (z = ZN). To reduce the effect of the insignificant warming at depths per water profile, we define the MHW depth as the depth where a fraction (ε=0.95) of the cumulative Ta is reached:
Based on MHW depth values, Ta profiles were then divided into three categories: Category 1 (shallow, 0–150 m), Category 2 (intermediate, 150–700 m), and Category 3 (deep, >700 m). It is noteworthy that, within the SAP area, float profiles categorized as Categories 2 and 3 consistently exhibit no negative temperature anomalies. However, they are classified into these categories based on their respective depths, shallower or deeper than 700 m. Additionally, despite the limited number of profiles available in this region, they all fall within the cyclonic gyre. Hence, we are confident in considering them as representative of the entire SAP region. The median profile () for each category was obtained by spatially averaging all the available data in the different sectors in the warm period using 2022 and FLOAT climatology Argo data. Considering that the 2022 MHW extends until the spring of 2023 (Marullo et al., 2023), the median profiles for the fall period were also examined to investigate the accumulation of the heat in the water column. The mean Ta values averaged in the surface, intermediate, and deep layers as well as other additional information (number of profiles, MHW depth, max Ta, and depth of max) are listed in Table 2.
Lastly, the Brunt–Väisälä frequency squared (N2) for the year 2022 and in the upper 150 m depth was computed using monthly averaged temperature and salinity Argo float profiles for each sector in order to support the vertical heat penetration. The same procedure was adopted to calculate the N2 anomaly with respect to the FLOAT climatology.
Table 2Characteristics of the 2022 MHW in Category 1 (C1), Category 2 (C2), and Category 3 (C3): MHW depth, surface temperature anomaly (“Surface”), maximum temperature anomaly (“Max”), and the depth where it occurs (“Depth of max”). Also shown are mean temperature anomaly values for the surface (0–150 m), intermediate (150–700 m), and deep (700–2000 m) layers for each category and for the FLOAT climatology (“clim.”).
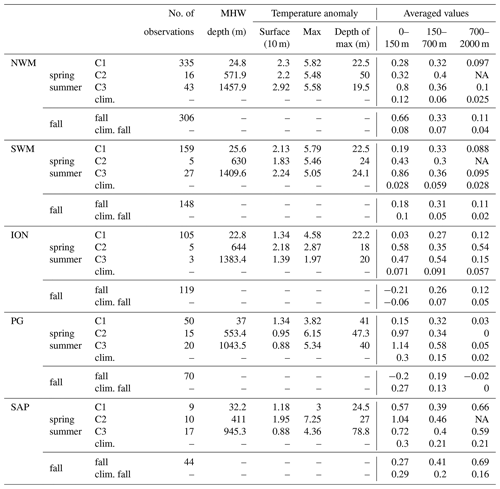
NA – not available.
In the surface layer, the OHCA2022 displayed inhomogeneous warming patterns, with positive anomaly areas adjacent to others with strong negative anomalies (Fig. 1c). Largest positive anomalies were observed in the western Mediterranean, southern Adriatic, eastern Ionian, and northern Levantine basins. In the intermediate and deep layers, the warming was more homogeneous and widespread (Fig. 1d, e), where the majority of bins showed positive values of OHCA2022; specifically, the western and central Mediterranean areas along with the Aegean Sea showed a more pronounced warming compared to the Levantine basin, which exhibits a slight cooling in some bins of the central and eastern sectors. It can be stated that half of this warming in the intermediate and deep layers is due to the 2022 MHW, while the other half is due to the long-term warming of the ocean. This consideration stems from comparing the current OHCA2022 with OHC trends defined by Kubin et al. (2023). To perform this study, five regions (NWM, SWM, ION, SAP, and PG; colored boxes in Fig. 1b) were selected. This choice was motivated by the highest 2022 sea surface temperature (SST) anomaly registered in the band from 0 to 25° E (Marullo et al., 2023) and by the availability of float data in both May–September and October–December 2022 periods. Figure 2 shows profiles for the warm season of each sector for each MHW depth category and for the FLOAT climatology.
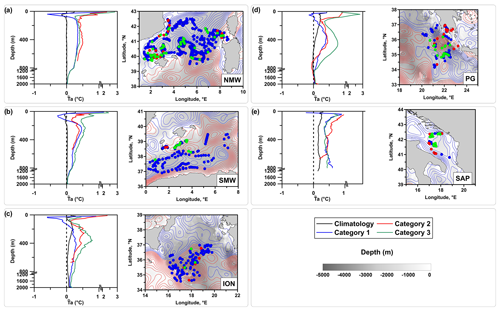
Figure 2(a–c, left) Median profiles of temperature anomaly computed for each sector (NWM, SWM, ION, PG, SAP) and for the 2022 warm season (May–September) using Argo float data with respect to the 1985–2018 SDC climatology dataset. Black lines highlight the FLOAT climatology profiles, while blue, red, and green profiles indicate shallow (0–150 m), intermediate (150–700 m), and deep (>700 m) categories, respectively. (d, e, right) Positive and negative contours of the absolute dynamic topography with 1 cm spacing are displayed by red and blue lines, while the colored dots are associated with the float positions of each category.
In the NWM and SWM sectors, the circulation is strongly influenced by the presence of two intense and permanent currents (Fig. 1a): the southwestward Northern Current (Poulain et al., 2012; Escudier et al., 2021) and the eastward along-slope Algerian Current (which transports waters of Atlantic origin in the upper water column; Poulain et al., 2021) in the NWM and in the SWM, respectively. Therefore, float profiles were mainly located along the boundary of cyclonic circulations as highlighted by the absolute dynamic topography (product ref. no. 3, Table 1; Fig. 2a, b). In the ION sector, float profiles were mainly distributed in the anticyclonic meander of the Mid-Ionian Jet (Fig. 2c), a strong meandering current that, together with the Atlantic–Ionian Stream (AIS), transports Atlantic Water from the western to the eastern Mediterranean Sea (Poulain et al., 2012, 2013; Menna et al., 2019a; Fig. 1a). Although the NWM, SWM, and ION sectors have different oceanographic characteristics, they showed a similar response to the 2022 MHW (Fig. 2a–c). Most profiles belong to Category 1, and the mean MHW depth falls into the 20–25 m layer (Table 2). Profiles, characterized by shallow MHW penetration (blue lines in Fig. 2a–c), showed a decreasing warming in the first 50 m with the maximum close to the surface (22.2–22.5 m; Table 2). The layer between 50 and 100 m depth showed a negative with maxima of −0.65, −0.2, and −0.53 °C at 50, 70, and 40 m depth, in the NWM, SWM, and ION sectors, respectively (Fig. 2a–c). The median profiles derived from the FLOAT climatology (black lines in Fig. 2a–c) do not exhibit this negative anomaly (or only to a very small extent), suggesting, therefore, a possible link between this behavior and the occurrence of the 2022 MHW. Below 100 m depth, becomes positive again with mean values of ∼ 0.3 °C in the intermediate layer and values lower than 0.12 °C in the deep layer. Profiles characterized by intermediate MHW penetration (red lines in Fig. 2a–c; MHW depth between 570 and 650 m, Table 2) were located in coastal areas of the western Mediterranean and in frontal zones in the ION sector and showed positive throughout the water column, with values in the range of 0.3–0.6 °C. Profiles, characterized by deep MHW penetration (green lines in Fig. 2a–c; MHW depth ∼ 1400 m, Table 2), showed the largest in the surface layer in the two sectors of the western Mediterranean (>0.8 °C), while the ION sector depicted the largest anomalies in the intermediate layer (>0.5 °C). These results are consistent with the warming trend of the western Mediterranean Sea over the last 15 years of 0.09 ± 0.02 °C yr−1 and 0.03 ± 0.01 °C yr−1 for surface waters and intermediate waters, respectively (Kubin et al., 2023).
The PG is located on the eastern side of the northern Ionian Sea, southwest of the Peloponnese coast (Fig. 1a). It is a subbasin anticyclonic feature (diameter of ∼ 120 km; Pinardi et al., 2015) that extends from the surface down to 800–1000 m depth (Malanotte-Rizzoli et al., 1997; Kovačević et al., 2015), and it is forced by the Etesian winds (Ayoub et al., 1998; Mkhinini et al., 2014; Menna et al., 2021). In the late summer to fall, the Etesian winds amplify their acceleration and wind shear in the region of the western Cretan straits (Mkhinini et al., 2014); therefore, larger anticyclonic vorticities are observed during these months in the PG region (Menna et al., 2019a). In the PG sector, profiles for the three categories showed positive temperature anomalies in the first 800 m of the water column, which coincides with the vertical extension of the gyre itself (Fig. 2d). Profiles that fall into Category 1 showed a decreasing warming in the first 70 m, anomaly values close to zero in the 70–150 m layer, and increasing warming in the 150–400 m layer. The mean anomaly in the intermediate layer of Category 1 is 0.3 °C (Table 2). Category 2 profiles were retrieved mainly in the coastal area near the Peloponnese, while Category 3 profiles were found within the gyre area. Categories 2 and 3 showed a strong warming in the surface layer (0.97 and 1.14 °C, respectively), a mean warming in the range of 0.3–0.6 °C in the intermediate layer and no warming compared to the SDC climatology was observed in the deep layer (Table 2).
The SAP is one of the sites of open ocean convection in the Mediterranean Sea, characterized by a complex thermohaline circulation that influences the physical and biogeochemical properties of the dense waters formed in its interior and the strength of winter convection (Martellucci et al., 2024; Di Biagio et al., 2023; Menna et al., 2022, OSR6; Pirro et al., 2022). This sector showed positive temperature anomalies in all layers and in all categories (Fig. 2d). Most profiles belong to Category 3 with a mean MHW depth of ∼ 950 m and maximum at ∼ 80 m depth. The largest mean warming was observed in the surface layer of each category (0.6–1.04 °C) followed by the deep layer that had an exceptional warming of ∼ 0.6 °C and finally by the intermediate layer that had a mean warming of ∼ 0.4 °C (Table 2).
All five sectors showed a larger warming than the FLOAT climatology with a mean temperature increase in the 2022 warm season between 0.2 and 0.8 °C in response to the MHW event (Table 2). Some differences in warming observed among the sectors are related to their peculiar hydrological and dynamical characteristics. During the warm season, the surface layer of the NWM and SWM sectors and partially of the ION sector was characterized by both larger stratifications and stratification anomalies compared to the FLOAT climatology (Fig. 3a, b). Strong stratification prevents vertical heat penetration, causing negative in the 50–100 m layer (Fig. 2a–c). In the PG sector, the warm-season stratification anomaly was consistent with the climatology (Fig. 3b), and vertical heat penetration was closely related to the gyre dynamics. In the SAP sector, stratification during the warm period was lower than the climatology, suggesting an instability of the water column and, therefore, of the transport of the vertical heat to the deep layers. The median of all profiles available in the 2022 warm season, when not categorized, closely aligns with the median of profiles in Category 1 (Fig. 3c, d). This condition arises because Category 1 consistently boasts the highest number of profiles across various sectors.
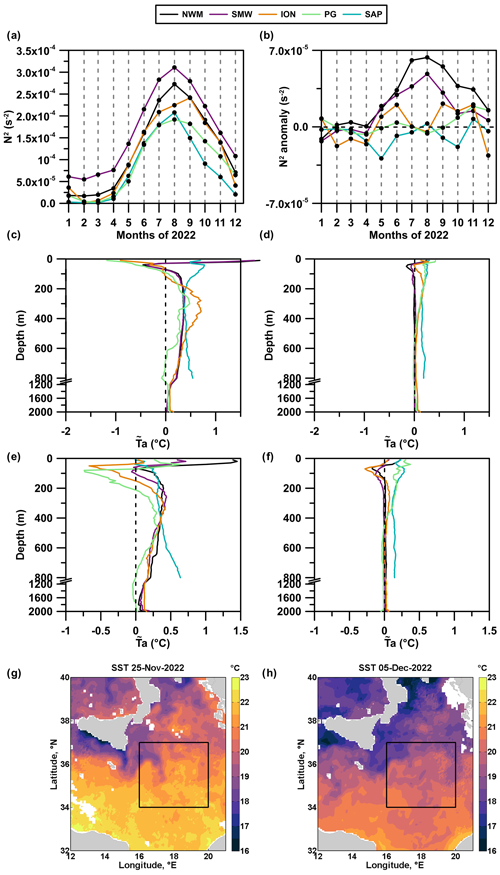
Figure 3(a) Monthly averaged Brunt–Väisälä frequency squared (N2) computed in the surface layer (0–150 m) using 2022 Argo float data. (b) Monthly averaged Brunt–Väisälä frequency squared anomaly (N2 anomaly) computed in the surface layer with respect to the FLOAT climatology. (c) Median temperature anomaly (°C) computed in the warm season (May–September) from Argo float profiles in 2022 and (d) in 2001–2018 with respect to the SDC climatology. (e) Median temperature anomaly (°C) computed in the fall period (October–December) from Argo float profiles in 2022 and (f) in 2001–2018 with respect to the SDC climatology. Daily sea surface temperature (°C) in the ION sector (black box) for (g) late November 2022 and (h) early December 2022.
Larger warming of the water column was observed in fall 2022 compared to the SDC climatology in all sectors, except for the surface layer of the ION and PG sectors (Fig. 3e). The stronger warm-season stratification observed in the NWM and SWM sectors (Fig. 3a, b) corresponds to enhanced vertical heat propagation in the surface and intermediate layers in fall 2022 (Fig. 3e, Table 2). Negative values in the surface layer of the ION sector were attributed to an upwelling event along the southern coast of Sicily between November and December 2022 as shown by the sea surface temperature (product ref. no. 2, Table 1; Fig. 3g, h). The northern part of the Sicily Channel is an area of strong eddy kinetic energy (Poulain et al., 2012), influenced by Ekman transport and advection of waters from the western to the eastern Mediterranean (Molcard et al., 2002; Falcini and Salusti, 2015; Schroeder et al., 2017; Menna et al., 2019b). The cold waters upwelled off the southern coast of Sicily in November 2022 (Fig. 3g) were advected to the Ionian Sea through the Atlantic–Ionian Stream and the Mid-Ionian Jet pathways (Fig. 1a), gradually cooling the waters in the ION sector (Fig. 3h). The negative anomaly in the surface layer of the ION sector is not only limited to 2022 but is also a permanent characteristic of the area related to the upwelling phenomena, as confirmed by the profile derived from the FLOAT climatology (orange line in Fig. 3f) and by trends in the OHC anomaly estimated by Dayan et al. (2023) over the period 1987–2019. Negative values in the PG sector were attributable to the typical downwelling process of this region that is associated with the gyre dynamics. The downwelling contributed to the vertical propagation of the 2022 MHW, with a strong spring–summer warming in the first 800 m of the water column (Fig. 2d), keeping the stratification values similar to the FLOAT climatology (no significant increases in N2 anomaly were registered due to the 2022 heat wave; Fig. 3b). In this way, fall cooling can penetrate deep into the water column, therefore causing negative values in the surface layer (Fig. 3e; Table 2).
In recent years, the SAP has been experiencing a significant temperature increase in the deep layer (trend of ∼ 0.06 °C yr−1 in the 2013–2020 period according to Kubin et al., 2023) and salinity increase in the surface and intermediate layers (Martellucci et al., 2024; Menna et al., 2022, OSR6; Mihanović et al., 2021), with possible future impacts on the entire thermohaline circulation of the Eastern Mediterranean. It is of general understanding that convection sites contribute to the propagation of the MHW signal from the surface to the subsurface interior of the water column (Dayan et al., 2023; Kubin et al., 2023), but specific analyses at the local scale are not yet available (Juza et al., 2022). Our results show a fairly significant warming of the SAP in both spring–summer (Figs. 2e and 3c) and fall 2022 (light blue line in Fig. 3f) and a significant positive anomaly of the FLOAT climatology compared to the SDC one (black line in Fig. 2e and light blue line in Fig. 3f). In fall, the largest values in the SAP were observed in the deep layer (∼ 0.69 °C; Table 2, Fig. 3e). Mean profiles derived from the FLOAT climatology (black line in Fig. 2e and light blue line in Fig. 3f) showed positive values compared to the SDC one, confirming the warming trend throughout the water column over the past decade. Beyond the impact of global warming on the Mediterranean Sea, the 2022 MHW led to an additional heating in the SAP, which was transferred to the deeper layers and favored by dynamical features of this area.
This study aimed to characterize the 2022 MHW in the subsurface layers and attempted to explain the mechanisms that drive the heat penetration to deep layers. However, further and more detailed investigations are needed to better support this last point. We showed that the effects of the 2022 MHW were felt in all layers of the Mediterranean Sea with vertical heat propagation extending from the surface to ∼ 1500 m depth. In the surface layer, heat penetration and storage are related to the strength of the stratification and/or advection from adjacent regions. In contrast, the transport and the storage of heat in the intermediate and deep layers are closely linked to the dynamics of each area. These considerations are in line with the findings by Elzahaby et al. (2021) and Zhang et al. (2023), who noted that shallower MHWs are primarily influenced by surface air–sea fluxes, whereas deeper MHWs are predominantly driven by advection, manifesting distinct dynamics across various oceanic regions.
In the western Mediterranean and western Ionian Sea sectors, heat is mainly stored in the surface layer (shallow MHW depths and stronger stratification), so this layer is significantly warmer than the climatology even during the following fall. Although deep MHW penetration in these regions is limited to coastal and frontal/eddy zones, it reaches the higher MHW depth estimated during the event. Sectors characterized by specific dynamics (downwelling, convection) quickly distribute the heat in the water column, even during the event. Intermediate layers exhibit comparable heating both during and after the MHW event, implying that heat can be stored there for extended periods and can be regarded as a long-term signal.
The warming signal in the intermediate and deep layers could also be influenced by heat advection from adjacent basins; however, we are aware that this topic needs to be studied in more detail in the future. In this context, the use of two climatologies and the cumulative anomaly threshold in the present analysis should have eliminated most of the signal associated with the ocean warming trend and advection; therefore, the additional warming registered in spring–summer 2022 compared to the FLOAT climatology can be attributed to the effects of the 2022 MHW along the entire water column. Further studies are needed to investigate the effects that this warming may have on the physical and biological oceanic processes, with implications for the thermohaline circulation of the entire Mediterranean Sea.
The codes and data are available upon request to Annunziata Pirro (apirro@ogs.it). Further information can also be found in Table 1.
AP, MM, and RM conceptualized the study. AP and MM prepared the original manuscript. AP, MM, RM, EM, AG, GN, EK, and MJ reviewed and edited the manuscript. AP, MM, and RM created the methodology. AP, MM, RM, and EK created the codes and performed the formal analysis. AP, MM, and RM conducted the investigation. AG, AB, and MP curated the data. EM was in charge of the Argo-Italy infrastructure management and funding acquisition. All authors have read and agreed to the published version of the paper.
The contact author has declared that none of the authors has any competing interests.
The Copernicus Marine Service offering is regularly updated to ensure it remains at the forefront of user requirements. In this process, some products may undergo replacement or renaming, leading to the removal of certain product IDs from the catalogue.
If readers have any questions or require assistance regarding these modifications, please feel free to reach out to the Copernicus Marine Service user support team for further guidance. They will be able to provide the necessary information to address concerns and find suitable alternatives.
Publisher's note: Copernicus Publications remains neutral with regard to jurisdictional claims made in the text, published maps, institutional affiliations, or any other geographical representation in this paper. While Copernicus Publications makes every effort to include appropriate place names, the final responsibility lies with the authors.
This paper was edited by Gilles Garric and reviewed by two anonymous referees.
Argo: Argo float data and metadata from Global Data Assembly Centre (Argo GDAC), SEANOE, https://doi.org/10.17882/42182, 2023.
Ayoub, N., Le Traon, P.-Y., and De Mey, P.: A description of the Mediterranean surface variable circulation from combined ERS-1 and TOPEX/POSEIDON altimetric data, J. Marine Syst., 18, 3–40, https://doi.org/10.1016/S0924-7963(98)80004-3, 1998.
Bensoussan, N., Chiggiato, J., Buongiorno Nardelli, B., Pisano, A., and Garrabou, J.: Insights on 2017 marine heat waves in the Mediterranean sea, J. Oper. Oceanogr., 12, s26–s30, https://doi.org/10.1080/1755876X.2019.1633075, 2019.
Darmaraki, S., Somot, S., Sevault, F., and Nabat, P.: Past variability of Mediterranean Sea marine heatwaves, Geophys. Res. Lett., 46, 9813–9823, https://doi.org/10.1029/2019GL082933, 2019.
Dayan, H., McAdam, R., Juza, M., Masina, S., and Speich, S.: Marine heat waves in the Mediterranean Sea: An assessment from the surface to the subsurface to meet national needs, Front. Mar. Sci., 10, 1045138, https://doi.org/10.3389/fmars.2023.1045138, 2023.
Di Biagio, V., Martellucci, R., Menna, M., Teruzzi, A., Amadio, C., Mauri, E., and Cossarini, G.: Dissolved oxygen as an indicator of multiple drivers of the marine ecosystem: the southern Adriatic Sea case study, in: 7th edition of the Copernicus Ocean State Report (OSR7), edited by: von Schuckmann, K., Moreira, L., Le Traon, P.-Y., Grégoire, M., Marcos, M., Staneva, J., Brasseur, P., Garric, G., Lionello, P., Karstensen, J., and Neukermans, G., Copernicus Publications, State Planet, 1-osr7, 10, https://doi.org/10.5194/sp-1-osr7-10-2023, 2023.
Elzahaby, Y. and Schaffer, A.: Observational insight into the subsurface anomalies of marine heatwaves, Front. Mar. Sci., 6, 745, https://doi.org/10.3389/fmars.2019.00745, 2019.
Elzahaby, Y., Schaeffer, A., Roughan, M., and Delaux, S.: Oceanic circulation drives the deepest and longest marine heatwaves in the East Australian Current system, Geophys. Res. Lett., 48, e2021GL094785, https://doi.org/10.1029/2021GL094785, 2021.
Escudier, R., Clementi, E., Cipollone, A., Pistoia, J., Drudi, M., Grandi, A., Lyubartsev, V., Lecci, R., Aydogdu, A., Delrosso, D., Omar, M., Masina, S., Coppini, G., and Pinardi, N.: A High Resolution Reanalysis for the Mediterranean Sea, Front. Earth Sci., 9, 702285, https://doi.org/10.3389/feart.2021.702285, 2021.
Escudier, R., Clementi, E., Nigam, T., Aydogdu, A., Fini, E., Pistoia, J., Grandi, A., and Miraglio, P.: EU Copernicus Marine Service Quality Information Document for Mediterranean Sea Physics Reanalysis, MEDSEA_MULTIYEAR_PHY_006_004, Issue 2.3, Mercator Ocean International, https://catalogue.marine.copernicus.eu/documents/QUID/CMEMS-MED-QUID-006-004.pdf (last access: 19 May 2023), 2022.
EU Copernicus Marine Service Product: Mediterranean Sea – In-Situ Near Real Time Observations, Mercator Ocean International [data set], https://doi.org/10.48670/moi-00044, 2022a.
EU Copernicus Marine Service Product: Mediterranean Sea Physics Reanalysis, CMCC Foundation [data set], https://doi.org/10.25423/CMCC/MEDSEA_MULTIYEAR_PHY_006_004_E3R1I, 2022b.
EU Copernicus Marine Service Product: European Seas Gridded L 4 Sea Surface Heights And Derived Variables Nrt, Mercator Ocean International [data set], https://doi.org/10.48670/moi-00142, 2023.
Falcini, F. and Salusti, E.: Friction and mixing effects on potential vorticity for bottom current crossing a marine strait: an application to the Sicily Channel (central Mediterranean Sea), Ocean Sci., 11, 391–403, https://doi.org/10.5194/os-11-391-2015, 2015.
Galli, G., Solidoro, C., and Lovato, T.: Marine heat waves hazard 3D maps and the risk for low motility organisms in a warming Mediterranean Sea, Front. Mar. Sci., 4, 136, https://doi.org/10.3389/fmars.2017.00136, 2017.
Garrabou, J., Gómez-Gras, D., Medrano, A., et al.: Marine heatwaves drive recurrent mass mortalities in the Mediterranean Sea, Glob. Change Biol., 28, 5708–5725, https://doi.org/10.1111/gcb.16301, 2022.
Hobday, A. J., Alexander, L. V., Perkins, S. E., Smale, D. A., Straub, S. C., Oliver, E. C., Benthuysen, J. A., Burrows, M. T., Donat, G. M., Feng, M., Holbrook, N., J., Moore, P. J., Scannel, H. A., Gupta, A. S., and Wernberg, T.: A hierarchical approach to defining marine heatwaves, Prog. Oceanogr., 141, 227–238, https://doi.org/10.1016/j.pocean.2015.12.014, 2016.
Holbrook, N. J., Gupta, A. S., Oliver, E. C., Hobday, A. J., Benthuysen, J. A., Scannell, H. A., Smale, D. A., and Wernberg, T.: Keeping pace with marine heatwaves, Nat. Rev. Earth Env., 1, 482–493, https://doi.org/10.1038/s43017-020-0068-4, 2020.
Hu, S., Li, S., Zhang, Y., Guan, C., Du, Y., Feng, M., Ando, K., Wang, F., Schiller, A., and Hu, D.: Observed strong subsurface marine heatwaves in the tropical western Pacific Ocean, Environ. Res. Lett., 16, 104024, https://doi.org/10.1088/1748-9326/ac26f2, 2021.
Ibrahim, O., Mohamed, B., and Nagy, H.: Spatial variability and trends of marine heat waves in the Eastern Mediterranean Sea over 39 years, J. Mar. Sci. Eng., 9, 643, https://doi.org/10.3390/jmse9060643, 2021.
In Situ TAC partners: EU Copernicus Marine Service Product User Manual for Mediterranean Sea – In-Situ Near Real Time Observations, INSITU_MED_PHYBGCWAV_DISCRETE_MYNRT_013_035, Issue 1.14, Mercator Ocean International, https://catalogue.marine.copernicus.eu/documents/PUM/CMEMS-INS-PUM-013-030-036.pdf (last access: 19 May 2023), 2022.
IPCC (Intergovernmental Panel on Climate Change): Summary for Policymakers, in: Climate Change 2023: Synthesis Report. Contribution of Working Groups I, II and III to the Sixth Assessment Report of the Intergovernmental Panel on Climate Change, edited by: Core Writing Team, Lee, H., and Romero, J., IPCC, Geneva, Switzerland, 1–34, https://doi.org/10.59327/IPCC/AR6-9789291691647.001, 2023.
Juza, M., Fernández-Mora, A., and Tintoré, J.: Sub-Regional marine heat waves in the Mediterranean Sea from observations: long-term surface changes, subsurface and coastal responses, Front. Mar. Sci., 9, 785771, https://doi.org/10.3389/fmars.2022.785771, 2022.
Kovačević, V., Ursella, L., Gačić, M., Notarstefano, G., Menna, M., Bensi, M., and Poulain, P.-M.: On the Ionian thermohaline properties and circulation in 2010-2013 as measured by Argo floats, Acta Adriat., 56, 97–114, 2015.
Kubin, E., Menna, M., Mauri, E., Notarstefano, G., Mieruch, S., and Poulain, P.-M.: Heat content and temperature trends in the Mediterranean Sea as derived from Argo float data, Front. Mar. Sci., 10, 1271638, https://doi.org/10.3389/fmars.2023.1271638, 2023.
Lecci, R., Drudi, M., Grandi, A., Cretì, S., and Clementi, E.: EU Copernicus Marine Service Product User Manual for For Mediterranean Sea Physics Reanalysis, MEDSEA_MULTIYEAR_PHY_006_004, Issue 2.3, Mercator Ocean International, https://catalogue.marine.copernicus.eu/documents/PUM/CMEMS-MED-PUM-006-004.pdf (last access: 19 May 2023), 2022.
Liquete, C., Piroddi, C., Macías, D., Druon, J. N., and Zulian, G.: Ecosystem services sustainability in the Mediterranean Sea: assessment of status and trends using multiple modelling approaches, Sci. Rep., 6, 1–14, https://doi.org/10.1038/srep34162, 2016.
Malanotte-Rizzoli, P., Manca, B. B., Ribera D'Alcalà, M., Theocharis, A., Bergamasco, A., Bregant, D., Budillon, G., Civitarese, G., Georgoupoulos, D., Michelato A., Sansone, E., Scarazzato, P., Souvermezoglou, E.: A synthesis of the Ionian Sea hydrography, circulation and water masses pathways during POEM-Phase I, Prog. Oceanogr., 39, 153–204, https://doi.org/10.1016/S0079-6611(97)00013-X, 1997.
Martín-López, B., Oteros-Rozas, E., Cohen-Shacham, E., Santos-Martín, F., Nieto-Romero, M., Carvalho-Santos, C., González, J. A., García-Llorente, M., Klass, K., Geijzendorffer, I., Montes, C., and Cramer, W.: Ecosystem services supplied by Mediterranean Basin ecosystems, in: Routledge handbook of ecosystem services, 405–414, Routledge, ISBN 9781315775302, 2016.
Martellucci, R., Menna, M., Mauri, E., Pirro, A., Gerin, R., de Mendoza, F. P., Garić, R., Batistić, M., di Biagio, V., Giordano, P., Langone, L., Miserocchi, S., Gallo, A., Notarstefano, G., Savonitto, G., Bussani, A., Pacciaroni, M., Zuppelli, P., and Poulain, P. M.: Recent changes of the dissolved oxygen distribution in the deep convection cell of the southern Adriatic Sea, J. Marine Syst., 245, 103988, https://doi.org/10.1016/j.jmarsys.2024.103988, 2024.
Martínez, J., Leonelli, F. E., García-Ladona, E., Garrabou, J., Kersting, D., Bensoussan, N., and Pisano, A.: Evolution of marine heatwaves in warming seas: the Mediterranean Sea case study, Front. Mar. Sci., 10, 1193164, https://doi.org/10.3389/fmars.2023.1193164, 2023.
Marullo, S., Serva, F., Iacono, R., Napolitano, E., di Sarra, A., Meloni, D., Monteleone, F., Sferlazzo, D., De Silvestri L., de Toma, V., Pisano, A., Bellacicco, M., Landolfi, A., Organelli, E., Yang, C., and Santoleri, R.: Record-breaking persistence of the 2022/23 marine heatwave in the Mediterranean Sea, Environ. Res. Lett., 18, 114041, https://doi.org/10.1088/1748-9326/ad02ae, 2023.
Menna, M., Suarez, N. R., Civitarese, G., Gačić, M., Rubino, A., and Poulain, P. M.; Decadal variations of circulation in the Central Mediterranean and its interactions with mesoscale gyres, Deep-Sea Res. Pt. II, 164, 14–24, https://doi.org/10.1016/j.dsr2.2019.02.004, 2019a.
Menna, M., Poulain, P. M., Ciani, D., Doglioli, A., Notarstefano, G., Gerin, R., Rio, M. H., Santoleri, R., Gauci, A., and Drago, A.: New Insights of the Sicily Channel and Southern Tyrrhenian Sea Variability, Water, 11, 1355, https://doi.org/10.3390/w11071355, 2019b.
Menna, M., Gerin, R., Notarstefano, G., Mauri, E., Bussani, A., Pacciaroni, M., and Poulain, P. M.: On the circulation and thermohaline properties of the Eastern Mediterranean Sea, Front. Mar. Sci., 8, 671469, https://doi.org/10.3389/fmars.2021.671469, 2021.
Menna, M., Martellucci, R., Notarstefano, G., Mauri, E., Gerin, R., Pacciaroni, M., Bussani, A., Pirro, A., and Poulain, P. M.: Record-breaking high salinity in the South Adriatic Pit in 2020, J. Oper. Oceanogr., s199–s205, https://doi.org/10.1080/1755876X.2022.2095169, 2022.
Mihanović, H., Vilibić, I., Šepić, J., Matić, F., Ljubešić, Z., Mauri, E., and Gerin, R.: Observation, preconditioning and recurrence of exceptionally high salinities in the Adriatic Sea, Front. Mar. Sci., 8, 672210, https://doi.org/10.3389/fmars.2021.672210, 2021.
Mills, K. E., Pershing, A. J., Brown, C. J., Chen, Y., Chiang, F. S., Holland, D. S., Lehuta, S., Nye, J. A., Sun, J. C., Thomas, A. C., and Wahle, R. A.: Fisheries management in a changing climate: lessons from the 2012 ocean heat wave in the Northwest Atlantic, Oceanography, 26, 191–195, https://doi.org/10.5670/oceanog.2013.27, 2013.
Molcard, A., Gervasio, L., Gria, A., Gasparini, G. P., Mortier, L., Ozgokmen, T. M.: Numerical investigation of the Sicily Channel dynamics: density currents and water mass advection, J. Marine Syst., 36, 219–238, https://doi.org/10.1016/S0924-7963(02)00188-4, 2002.
Mkhinini, N., Coimbra, A. L. S., Stegner, A., Arsouze, T., Taupier-Letage, I., and Beranger, K.: Long-lived mesoscale eddies in the Eastern Mediterranean Sea: analysis of 20 years of AVISO geostrophic velocities, J. Geophys. Res.-Oceans, 119, 8603–8626, https://doi.org/10.1002/2014JC010176, 2014.
Oliver, E. C., Donat, M. G., Burrows, M. T., Moore, P. J., Smale, D. A., Alexander, L. V., Benthuysen, J. A., Feng, M., Gupta, A. S., Hobday, A. J., Holbrook, N. J., Perkins-Kirkpatrick, S. E., Scannell, H. E., Straub, S. C., and Wernberg, T.: Longer and more frequent marine heatwaves over the past century, Nat. Commun., 9, 1324, https://doi.org/10.1038/s41467-018-03732-9, 2018.
Pastor, F. and Khodayar, S.: Marine heat waves: Characterizing a major climate impact in the Mediterranean, Sci. Total Environ., 861, 160621, https://doi.org/10.1016/j.scitotenv.2022.160621, 2023.
Pinardi, N., Zavatarelli, M., Adani, M., Coppini, G., Fratianni, C., Oddo, P., Simoncelli, S., Tonani, M., and Lyubartsev, V.: Mediterranean Sea large-scale low-frequency ocean variability and water mass formation rates from 1987 to 2007: a retrospective analysis, Prog. Oceanogr., 132, 318–332, https://doi.org/10.1016/j.pocean.2013.11.003, 2015.
Pirro, A., Mauri, E., Gerin, R., Martellucci, R., Zuppelli, P., and Poulain, P. M.: New insights on the formation and breaking mechanism of convective cyclonic cones in the South Adriatic Pit during winter 2018, J. Phys. Oceanogr., 52, 2049–2068, https://doi.org/10.1175/JPO-D-21-0108.1, 2022.
Poulain, P. M., Menna, M., and Mauri, E.: Surface geostrophic circulation of the Mediterranean Sea derived from drifter and satellite altimeter data, J. Phys. Oceanogr., 42, 973–990, https://doi.org/10.1175/JPO-D-11-0159.1, 2012.
Poulain, P. M., Bussani, A., Gerin, R., Jungwirth, R., Mauri, E., Menna, M., and Notarstefano, G.: Mediterranean surface currents measured with drifters: From basin to subinertial scales, Oceanography, 26, 38–47, https://doi.org/10.5670/oceanog.2013.03, 2013.
Poulain, P. M., Centurioni, L., Özgökmen, T., Tarry, D., Pascual, A., Ruiz, S., Mauri, E., Menna, M., and Notarstefano, G.: On the structure and kinematics of an Algerian Eddy in the southwestern Mediterranean Sea, Remote Sens., 13, 3039, https://doi.org/10.3390/rs13153039, 2021.
Pujol, M.-I.: EU Copernicus Marine Service Product User Manual for European Seas Gridded L 4 Sea Surface Heights And Derived Variables Nrt, SEALEVEL_EUR_PHY_L4_NRT_OBSERVATIONS_008_060, Issue 7.0, Mercator Ocean International, https://catalogue.marine.copernicus.eu/documents/PUM/CMEMS-SL-PUM-008-032-068.pdf (last access: 19 May 2023), 2022.
Pujol, M.-I., Taburet, G., and SL-TAC team: EU Copernicus Marine Service Quality Information Document for European Seas Gridded L 4 Sea Surface Heights And Derived Variables Nrt, SEALEVEL_EUR_PHY_L4_NRT_OBSERVATIONS_008_060, Issue 8.2, Mercator Ocean International, https://catalogue.marine.copernicus.eu/documents/QUID/CMEMS-SL-QUID-008-032-068.pdf (last access: 19 May 2023), 2023.
Santora, J. A., Mantua, N. J., Schroeder, I. D., Field, J. C., Hazen, E. L., Bograd, S. J., Sydeman, W. J., Wells, B. K., Calambokidis, J., Saez, L., Lawson, D., and Forney, K. A.: Habitat compression and ecosystem shifts as potential links between marine heatwave and record whale entanglements, Nat. Commun., 11, 1–12, https://doi.org/10.1038/ s41467-019-14215-w, 2020.
Scannell, H. A., Johnson, G. C., Thompson, L., Lyman, J. M., and Riser, S. C.: Subsurface evolution and persistence of marine heatwaves in the Northeast Pacific, Geophys. Res. Lett., 47, e2020GL090548, https://doi.org/10.1029/2020GL090548, 2020.
Schaeffer, A. and Roughan, M.: Subsurface intensification of marine heatwaves off southeastern Australia: the role of stratification and local winds, Geophys. Res. Lett., 44, 5025–5033, https://doi.org/10.1002/2017gl073714, 2017.
Schroeder, K., Chiggiato, J., Josey, S. A., Borghini, M., Aracri, S., and Sparnocchia, S.: Rapid response to climate change in a marginal sea, Sci. Rep., 7, 4065, https://doi.org/10.1038/s41598-017-04455-5, 2017.
Simon, A., Pires, C., Frölicher, T. L., and Russo, A.: Long-term warming and interannual variability contributions' to marine heatwaves in the Mediterranean, Weather and Climate Extremes, 42, 100619, https://doi.org/10.1016/j.wace.2023.100619, 2023.
Simoncelli, S., Oliveri, P., Mattia, G., Myroshnychenko, V., Barth, A., and Troupin, C.: SeaDataCloud Temperature and Salinity Climatology for the Mediterranean Sea (Version 2), Product Information Document (PIDoc), SeaDataCloud, https://doi.org/10.13155/77514, 2020a.
Simoncelli, S., Oliveri, P., and Mattia, G.: SeaDataCloud Mediterranean Sea – V2 Temperature and Salinity Climatology, Sextant [data set], https://doi.org/10.12770/3f8eaace-9f9b-4b1b-a7a4-9c55270e205a (last access: 19 May 2023), 2020b.
Smale, D. A., Wernberg, T., Oliver, E., Thomsen, M., Harvey, B. P., Straub, S. C., Burrows, M., Alexander, L., Benthuysen, J., Donat, M., Feng, M., Hobday, A., Holbrook, N., Perkins-Kirkpatrick, S., Scannell, H. A., Sen Gupta, A., Payne, B. L., and Moore, P.: Marine heatwaves threaten global biodiversity and the provision of ecosystem services, Nat. Clim. Change, 9, 306–312, https://doi.org/10.1038/s41558-019-0412-1, 2019.
Von Schuckmann, K., Palmer, M. D., Trenberth, K. E., Cazenave, A., Chambers, D., Champollion, N., Hansen, J., Josey, S. A., Loeb, N., Mathieu, P. P., Meyssignac, B., and Wild, M.: An imperative to monitor earth's energy imbalance, Nat. Clim. Change, 6, 138–144, https://doi.org/10.1038/nclimate2876, 2016.
Wehde, H., Schuckmann, K. V., Pouliquen, S., Grouazel, A., Bartolome, T., Tintore, J., De Alfonso Alonso-Munoyerro, M., Carval, T., Racapé, V., and the INSTAC team: EU Copernicus Marine Service Quality Information Document for Mediterranean Sea – In-Situ Near Real Time Observations, INSITU_MED_PHYBGCWAV_DISCRETE_MYNRT_013_035, Issue 2.2, Mercator Ocean International, https://catalogue.marine.copernicus.eu/documents/QUID/CMEMS-INS-QUID-013-030-036.pdf (last access: 19 May 2023), 2022.
Wernberg, T., Smale, D. A., Tuya, F., Thomsen, M. S., Langlois, T. J., De Bettignies, T., Bennet, S., and Rousseaux, C. S.: An extreme climatic event alters marine ecosystem structure in a global biodiversity hotspot, Nat. Clim. Change, 3, 78–82, https://doi.org/10.1038/nclimate1627, 2013.
Wong, A. P., Wijffels, S. E., Riser, S. C., et al.: Argo Data 1999–2019: Two Million Temperature-Salinity Profiles and Subsurface Velocity Observations From a Global Array of Profiling Floats, Front. Mar. Sci., 7, 700, https://doi.org/10.3389/fmars.2020.00700, 2020.
Zhang, Y., Du, Y., Feng, M., and Hobday, A. J.: Vertical structures of marine heatwaves, Nat. Commun., 14, 6483, https://doi.org/10.1038/s41467-023-42219-0, 2023.