the Creative Commons Attribution 4.0 License.
the Creative Commons Attribution 4.0 License.
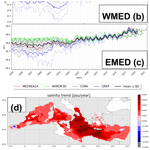
The dynamical role of upper layer salinity in the Mediterranean Sea
Pietro Miraglio
Romain Escudier
Emanuela Clementi
Simona Masina
The Mediterranean Sea (MED) is a semi-enclosed basin with an excess amount of evaporation compared to the water influx through precipitation at the surface and river runoff on the land boundaries. The deficit in the water budget is balanced by the inflow in the Strait of Gibraltar and Turkish Straits System connecting the Mediterranean with the less saline Atlantic Ocean and the Black Sea, respectively. There is evidence that the Mediterranean region is a hotspot in a warming climate, which will possibly change the water cycle significantly, but with large uncertainties. Therefore, it is inevitable to monitor the evolution of the essential ocean variables (EOVs) to respond to the associated risks and mitigate the related problems. In this work, we evaluate the evolution of the salinity content and anomaly between 0–300 m in the Mediterranean Sea during the last decades using the Copernicus Marine Service reanalysis and in situ objective analysis products. The results show an increasing mean salinity with a stronger trend in the eastern Mediterranean (EMED) basin. The spread of the products implies a larger variability in the western Mediterranean (WMED) basin, while the standard deviation is lower in the eastern side, especially in the Ionian and the Levantine basins.
- Article
(6123 KB) - Full-text XML
- BibTeX
- EndNote
The Mediterranean Sea (MED) is warming (Pisano et al., 2020). It is evaporating more and more (Skliris et al., 2018; Jordà et al., 2017) with marine heat waves increasing in intensity, duration and frequency (Juza et al., 2022; Dayan et al., 2022). The Mediterranean region is a hotspot with global warming (Tuel and El Tahir, 2020) that will likely alter the water cycle (Cos et al., 2022). Tracking the changes of the essential ocean variables (EOVs) is crucial in order to understand the impact of climate change. Two of these EOVs are linked to the ocean salinity at the surface and subsurface, which will be affected significantly by the surface heat and freshwater fluxes. The global water cycle modulating the ocean salinity is a key element of the Earth's climate (Cheng et al., 2020). In the Mediterranean Sea, freshwater fluxes through the land (rivers) and atmosphere (evaporation and precipitation) are balanced by two sea straits, namely Gibraltar and Dardanelles, from which the less saline Atlantic Ocean and Black Sea waters flow into the basin with an annual net inflow of 0.78±0.05 Sv (Soto-Navarro et al., 2010) and 0.05±0.04 Sv (Jarosz et al., 2013), respectively. These density contrasts contribute to the wind-driven circulation and generate a highly energetic anti-estuarine circulation (Cessi et al., 2014). The salinity of the Atlantic Waters (AW) entering through the Straight of Gibraltar is about 36.2 psu. The salinity of the Dardanelles Strait can vary significantly and it can be as low as 27 psu (Aydogdu et al., 2018; Sannino et al., 2017). Recently, Fedele et al. (2022) studied the characterisation of the Atlantic Waters and Levantine intermediate waters (LIW) from the Argo profiles in the last 20 years. Their conclusion is a clear salinification and warming trend which characterised both AW and LIW over the last 2 decades. Skliris et al. (2018) argue that the Mediterranean basin salinification is driven by changes in the regional water cycle rather than by changes in salt transports at the straits, as it is shown by the water mass transformation distribution in salinity coordinates. However, we will show that there is a bigger uncertainty compared to most of the basin in the radius of influence of both the Gibraltar and Dardanelles straits. In Sect. 2, the data and methods used in this study are presented. In Sect. 3, the results are shown and discussed, while in Sect. 4, the conclusions are drawn.
In this study, the Copernicus Marine Service global and regional reanalyses as well as observational gridded products are used to explore the role of the salinity variability in the 0–300 m depth among different estimates as well as temporal and spatial anomalies against a mean.
The Mediterranean ∘ resolution regional reanalysis (hereinafter, MEDREA24; Escudier et al., 2021) from the Copernicus Marine Service is used as a regional high-resolution product. In this work, MEDREA24 and its interim extension until the end of 2021 are included. Moreover, the ∘ resolution Global Reanalysis Ensemble Product (hereinafter, GREP) is also used. It consists of the global reanalysis from Mercator Ocean's GLORYS2V4 (Lellouche et al., 2013), UK MetOffice's GLOSEA5v13 (MacLachlan et al., 2014) using the Forecasting Ocean Assimilation Model (FOAM) system (Blockley et al., 2014), CMCC C-GLORSv7 (Storto et al., 2016) and ECMWF's ORAS5 (Zuo et al., 2017). A study on the ocean heat content and steric sea level representation in the GREP ensemble can be found in Storto et al. (2019a). A more general status of the global ocean reanalysis is reviewed in Storto et al. (2019b). The period covered by the GREP is 1993–2019.
As observational products, the gridded reconstructions of CORA (Szekely et al., 2019) and ARMOR3D (Guinehut et al., 2012) are adopted. Both datasets are available between 1993 and 2020. In the CORA, the objective analysis is performed on measurements' anomalies relative to a first guess provided by monthly climatology for different decades in the World Ocean Atlas 2013 to accurately reproduce the climate tendencies; it is interpolated and centred on the 15th of each month. Instead, in ARMOR3D, the first guess is adopted from World Ocean Atlas 2018. Both products use an objective analysis method proposed by Bretherton et al. (1976).
The investigation is performed in the entire Mediterranean Sea (MED) as well as in the eastern (EMED) and western (WMED) basins, which have very different characteristics. The salinity mean () is computed using Eq. (1) as the monthly volume (V) average of each product between 0 and 300 m depth, i.e. excluding the shelf areas close to the coast with a depth less than 300 m.
The mean of different products and their standard deviation are evaluated in the common period 1993–2019. Besides this time frame, the CORA and ARMOR3D time series are available until 2020, while the MEDREA24 time series is provided up to 2021 (last 6 months extended in interim mode).
The salinity anomalies are computed using Eq. (2). The difference of the salinity, S, with a reference salinity, Sref, is normalised by the depth of the water column, which is constant in our case with z2=300 m and z1=0 m.
Equation (2) is a modified version of the one proposed in Boyer et al. (2007), which uses the salinity as a proxy for the equivalent freshwater content. This method has been later adopted in various studies including, among the others, Holliday et al. (2020), with a density weight to account for baroclinic properties of the water column. The formulation in Boyer et al. (2007) is based on a reference salinity. As an example, mean values of a basin (Aagaard and Carmack, 1989) is a widely used choice for Sref in global freshwater content calculations. However, it is argued that since a reference value can be chosen arbitrarily, this would bring ambiguity (Schauer and Losch, 2019) in computing the equivalent freshwater content. Therefore, in this study, we propose to evaluate the salinity content and anomaly following Eq. (2) by choosing Sref as a monthly climatology of each dataset computed from each product separately between 1993 and 2014. This period is chosen to be consistent with the Ocean Monitoring Indicators produced previously in the Mediterranean Sea and other Copernicus Marine domains. Furthermore, the calculations are performed in the entire Mediterranean Sea (MED) as well as in its western (WMED) and eastern (EMED) subbasins, separated at the Sicily Strait. In Fig. 1, we present the monthly variation of the Sref as an example only, the one from MEDREA24, which shows a clear difference in the seasonality in the EMED and WMED, with a maximum in March and December, respectively. It is also evident that the Mediterranean monthly salinity reference shows a seasonal cycle much similar to the one of the eastern basin (but with different magnitude) characterised by lower salinity during the summer period and larger values at the end of the year.
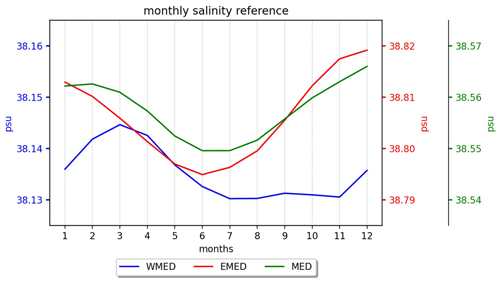
Figure 1The monthly reference salinity Sref estimates calculated from the MEDREA24 in the period 1993–2014. The green, blue and red curves show the MED, WMED and EMED regions, respectively on its corresponding vertical axis. The same calculation is done for each product separately (not shown) to evaluate Eq. (2) to compute salinity anomaly.
The Copernicus Marine Service products described in the previous section allow the assessment of the salinity content of the Mediterranean Sea along with its anomalies and trends during the last decades.
In Fig. 2, we present the time series of the mean salinity content in the first 300 m derived from the analysed products (MEDREA24 in red, GREP ensemble mean in blue, GREP ensemble members in thin light blue, CORA in dark green, ARMOR3D in light green) and their overall mean (in black) and spread (shaded grey).
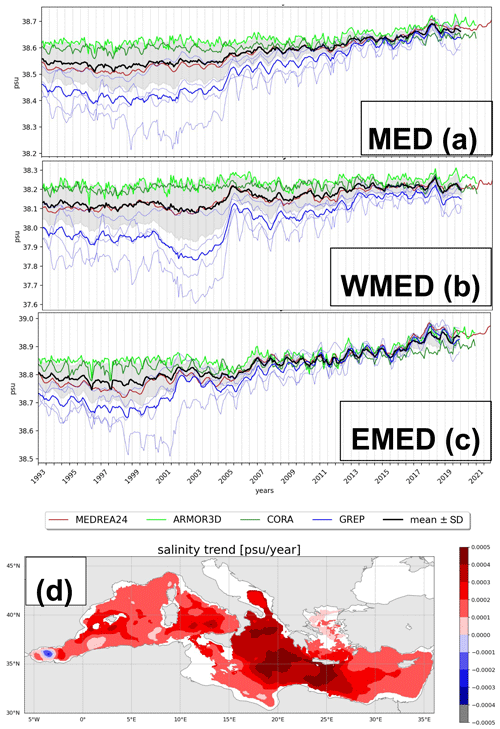
Figure 2Time series of mean salinity in the upper 300 m in the (a) entire Mediterranean Sea, (b) western Mediterranean basin and (c) eastern Mediterranean basin between the period 1993 and 2021 from MEDREA24, until the end of 2020 for ARMOR3D and CORA, and until the end of 2019 for GREP. The mean of all the products is drawn in black with their standard deviation shaded around the mean. The GREP ensemble members are depicted in thin blue curves. The GREP product covers the period until 2019, while the observational products CORA and ARMOR3D cover the period until 2020. The time series for MEDREA24 is extended until 2021 using the interim products. Panel (d) shows the salinity trend in the first 300 m from the MEDREA24 practical salinity unit (psu) per year.
During the early 1990s in the entire Mediterranean Sea (Fig. 2a), there is a large spread in salinity with the observations showing a higher salinity, while the reanalysis products present relatively lower salinity. This is the case until 2005. Coinciding with the global coverage of the Argo profilers in the early 2000s following the efforts in the Global Ocean Data Assimilation (GODAE) together with the Climate Variability and Predictability Programme (CLIVAR) and the Global Climate Observing System/Global Ocean Observing System (GCOS/GOOS), the spread among different products narrows. Possibly, the reanalyses are better constrained through data assimilation with this novel observation type (Johnson et al., 2022), which provides high-resolution and high-frequency temperature and salinity profiles all over the world's oceans, while the observation-based gridded products become more confident. The maximum spread between the period 1993–2019 occurs in the 1990s with a value of 0.096 psu, and it decreases to as low as 0.009 psu by the end of the 2010s. The mean salinity computed in the entire Mediterranean Sea from all products varies between approximately 38.5 and 38.6 psu with a spatiotemporal mean of 38.57 psu (Table 2).
Table 2The temporal mean salinity (in psu) and trend (in psu per year) in the 0–300 m between the common period 1993–2019 for separate products and their overall mean in Fig. 2.
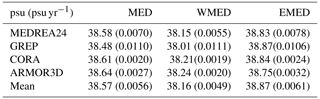
In the western Mediterranean (Fig. 2b), the overall mean is centred around 38.16 psu with a larger spread – with a maximum and minimum of 0.172 psu and 0.026, respectively – occurring in the early 2000s. An increase of the mean salinity in 2005 is evident from all the reanalysis products and, to a lesser extent, from the CORA dataset, for which one of the many possible reasons is the regime shift as discussed in Schroeder et al. (2016), corresponding to a major deep-water formation event at the beginning of the Western Mediterranean Transition (Zunino et al., 2012).
In the eastern Mediterranean (Fig. 2c), the overall mean is centred around 38.87 psu with a lower spread compared to the western basin, with a maximum and minimum of 0.086 psu and 0.003, respectively.
Overall, for the period between 1993–2019, we note that the observational products, gridded using optimal interpolation statistical techniques, show a higher average salinity compared to the reanalysis products that are dynamically integrated and corrected through data assimilation. The spread is representing the offset of the products more than their variability in the entire Mediterranean Sea, as well as in its eastern and western subdomains.
All the products show a positive trend between 1993–2019 (in parenthesis in Table 2). The trend in the mean of all products is calculated as 0.0056 psu yr−1. This trend is consistent with the estimates between 1950–2002 of Skliris et al. (2018) from the EN4 and MEDATLAS datasets, which show a trend of 0.0096 ± 0.0077 and 0.0088 ± 0.0092, respectively, in the first 150 m while showing a trend of 0.0067 ± 0.0040 and 0.0067 ± 0.0036 between 150–600 m. The differences in trend in different products that we used are mainly due to the discrepancies at the beginning of the time series. The weak consistency among the reanalyses visible during the first decade is likely due to the lack of observations not sufficient to constrain the different models, which use different physics and initialisation (e.g. Masina and Storto, 2017). The reduction of the spread among the products evolves in parallel to the increase of the observational coverage after the advent of the Argo network. The observational products will be impacted from the scarcity of the observations in the 1990s, since they rely on statistical methods. The trend calculated for each grid point from the MEDREA24, which is the analysed product covering the longest period, is presented in Fig. 2d. The dominant signal in the entire basin is positive with a larger amplitude in the Balearic Sea, Ionian Sea, Adriatic Sea, Western Levantine and with a less evident signal in the Gulf of Lions, Northern Aegean Sea and Eastern Levantine Basin. A small negative trend zone appears in the Alboran Sea. The trend in the entire analysed period is about 0.0049 psu yr−1 in the western basin. This is below the rate of the basin-wide trend, which is larger due to the trend in the eastern basin (0.0061 psu yr−1). Differences among different products, especially the objectively analysed observations and GREP, for the trend is larger in the western basin. They are more confined around the mean in the eastern basin, which may also explain the lower spread in this area as discussed below in Fig. 3b.
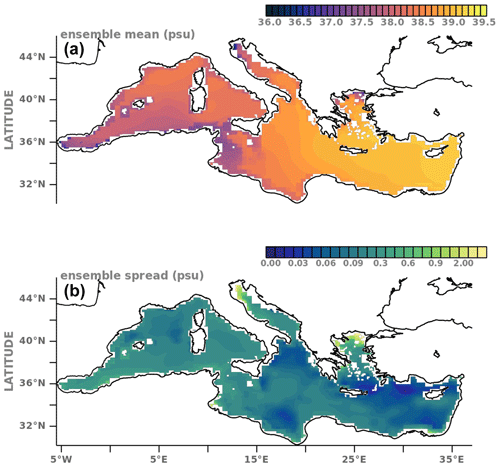
Figure 3The maps of the (a) mean and (b) spread of integrated salinity in the period between 1993–2014 in 0–300 m computed from the GREP ensemble mean, CORA, ARMOR3D and MEDREA24 products. We refer to the text for the information on the data products used. The analysis is performed only if the water column is deeper than 300 m. Note that in (b), the colour scale is not linear to show the smaller standard deviation.
For 2020, CORA and ARMOR3D products are available, and both continue to sustain the positive trend even though it is less evident in the western basin. Along with its interim extension, MEDREA24 shows an increasing mean salinity until the end of 2021. All products present larger values after 2016 and a maximum in 2018.
The spatial mean, computed between 1993–2014 from all products in the first 300 m (Fig. 3a), shows a gradual increase in the upper ocean integrated salinity from west to east. Minimum salinity occurs close to river mouths, such as in the North Adriatic Sea due to the freshwater input from the Po River, and on the pathways of the outflow of the Dardanelles and Gibraltar straits. The Atlantic Waters, modified through its route, can be traced till the eastern basin from its low salinity. The spread deduced from all the products (Fig. 3b) implies that they agree more, meaning lower spread, in the Levantine and Ionian Seas and to a lesser degree between the Balearic, Sardinia and Corsica islands. The spread is larger especially in the northern Aegean and Adriatic Sea and southwestern coast between the Gulf of Gabes and Strait of Gibraltar. This uncertainty or mismatch in the products is possibly due to the different volume fluxes through the rivers and straits.
In Fig. 4a–c, we show the time series of the salinity anomaly estimates in the western (Fig. 4a), eastern (Fig. 4b) and entire (Fig. 4c) basin from each product using Eq. (2). We recall that the salinity reference is computed for each product per se. Moreover, the salinity anomaly map in 2021 from MEDREA24 is depicted in Fig. 4d, computed against the overall mean between 1993–2014, which is shown in Fig. 3a.
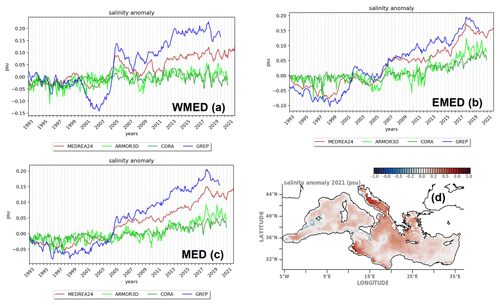
Figure 4Time series of the salinity anomaly from the MEDREA24, GREP, ARMOR3D and CORA products in the (a) western Mediterranean Sea, (b) eastern Mediterranean basin and (c) entire Mediterranean basin computed with respect to the monthly reference salinity estimates in the corresponding area in Fig. 1 calculated from the MEDREA24 in the period 1993–2014. The GREP products cover the period until 2019, while the observational products CORA and ARMOR3D cover the period until 2020. The time series for MEDREA24 is extended until 2021 using the interim products. Panel (d) shows the salinity anomaly in 2021 in the Mediterranean Sea against the mean of salinity in Fig. 3a.
The anomalies have a larger range in the reanalysis products. There is a negative anomaly within the first decade in GREP and MEDREA24 which turns to positive first in the western Mediterranean (Fig. 4a) and followed by the eastern basin (Fig. 4b) after 2006. In the CORA and ARMOR3D, instead, there is a clear increase in the salinity anomaly in the eastern Mediterranean and the entire basin with a less evident positive trend in the western basin. We summarise the mean salinity anomalies in Table 3.
Table 3The temporal mean salinity anomaly (in psu) in the 0–300 m between the common period 1993–2019 for separate products and their average in Fig. 3.
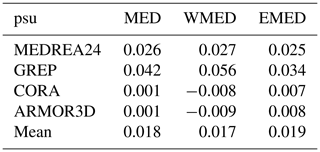
In 2021, the anomaly is mostly positive, with some negative anomaly structures on the path of the Atlantic Waters (Fig. 4d), Alboran Sea and upwelling favouring the Balearic Islands. Fedele et al. (2022) reports a positive salinity trend in the modified Atlantic and Levantine intermediate waters using 18-year-long (2001–2019) Argo profiles, which in general agrees with the anomaly map to a large extent. However, we note that the spread on the pathway of the water entering from the Strait of Gibraltar and reaching the Levantine basin has a relatively larger spread compared to the deeper areas (see Fig. 3b).
In this study, we presented the salinity characteristics of the Mediterranean Sea in the upper 300 m deduced from various products including reanalysis and gridded observational datasets released by the Copernicus Marine Service. The products with dynamically constructed ocean reanalysis and objectively analysed observations show significantly large spread at the beginning of the period of investigation, while the uncertainty is possibly reduced with the emergence of Argo profilers, which allowed wider spatial sampling and a higher frequency of sampling in the ocean. The mean salinity with its anomaly and trend is computed and analysed in the entire basin as well as in the western and eastern basins for all the datasets separately and averaged. The spatial maps of the mean and the spread of the salinity are depicted and discussed. The overall results show a salinification of the Mediterranean Sea agreeing with earlier studies (e.g. Skliris et al., 2018). The subbasin-scale investigation shows negative salinity anomalies in the western basin in the upwelling regions, which may imply stronger upwelling events, and waterway following the North African coast, which may be a consequence of the freshening North Atlantic water masses (Holliday et al., 2020). There is a large spread in the salinity estimates among different products, which is reduced with the introduction of the Argo profilers in the data assimilation components of the reanalysis systems. Besides the large spread, considering the reported discrepancies in the salinity measurements after 2016 (Barnoud et al., 2021), it is essential to use all available information sources for a more accurate state estimate and uncertainty quantification.
All datasets used in this article can be obtained from the Copernicus Marine Service catalogues as described in Table 1 with their names, temporal coverages and documentation.
AA and PM have done the investigation, developed the methodology and performed the data analysis. AA wrote the initial draft. All the authors reviewed and edited the paper.
The contact author has declared that none of the authors has any competing interests.
Publisher's note: Copernicus Publications remains neutral with regard to jurisdictional claims in published maps and institutional affiliations.
We would like to express our thanks to Katrin Schroeder and one anonymous referee for their useful comments, which helped us to improve the paper. We thank to Piero Lionello for his editorial suggestions. Finally, we would like to thank to Karina von Schuckmann for very useful discussions during course of the study.
This study has been conducted using EU Copernicus Marine Service Information. This work has been funded through the EU Copernicus Marine Med-MFC Service, lot no. 21002L5-COP-MFC MED-5500.
This paper was edited by Piero Lionello and reviewed by Katrin Schroeder and one anonymous referee.
Aagaard, K. and Carmack, E. C.: The role of sea ice and other fresh water in the Arctic circulation, J. Geophys. Res.-Oceans, 94, 14485–14498, 1989.
Aydoğdu, A., Pinardi, N., Özsoy, E., Danabasoglu, G., Gürses, Ö., and Karspeck, A.: Circulation of the Turkish Straits System under interannual atmospheric forcing, Ocean Sci., 14, 999–1019, https://doi.org/10.5194/os-14-999-2018, 2018.
Barnoud, A., Pfeffer, J., Guérou, A., Frery, M.-L., Siméon, M., Cazenave, A., Chen, J., Llovel, W., Thierry, V., Legeais, J. F., and Ablain, M.: Contributions of altimetry and Argo to non-closure of the global mean sea level budget since 2016, Geophys. Res. Lett., 48, e2021GL092824, https://doi.org/10.1029/2021GL092824, 2021.
Blockley, E. W., Martin, M. J., McLaren, A. J., Ryan, A. G., Waters, J., Lea, D. J., Mirouze, I., Peterson, K. A., Sellar, A., and Storkey, D.: Recent development of the Met Office operational ocean forecasting system: an overview and assessment of the new Global FOAM forecasts, Geosci. Model Dev., 7, 2613–2638, https://doi.org/10.5194/gmd-7-2613-2014, 2014.
Boyer, T., Levitus, S., Antonov, J., Locarnini, R., Mishonov, A., Garcia, H., and Josey, S. A.: Changes in freshwater content in the North Atlantic Ocean 1955–2006, Geophys. Res. Lett., 34, L16603, https://doi.org/10.1029/2007GL030126, 2007.
Bretherton, F. P., Davis, R. E., and Fandry, C. B.: A technique for objective analysis and design of oceanographic experiments applied to MODE-73, Deep-Sea Res., 23, 559–582, 1976.
Cessi, P., Pinardi, N., and Lyubartsev, V.: Energetics of semienclosed basins with two-layer flows at the strait, J. Phys. Ooceanogr., 44, 967–979, 2014.
Cheng, L., Trenberth, K. E., Gruber, N., Abraham, J. P., Fasullo, J. T., Li, G., Mann, M. E., Zhao, X., and Zhu, J.: Improved Estimates of Changes in Upper Ocean Salinity and the Hydrological Cycle, J. Climate, 33, 10357–10381, https://doi.org/10.1175/JCLI-D-20-0366.1, 2020.
Cos, J., Doblas-Reyes, F., Jury, M., Marcos, R., Bretonnière, P.-A., and Samsó, M.: The Mediterranean climate change hotspot in the CMIP5 and CMIP6 projections, Earth Syst. Dynam., 13, 321–340, https://doi.org/10.5194/esd-13-321-2022, 2022.
Dayan, H., McAdam, R., Masina, S., and Speich, S.: Diversity of marine heatwave trends across the Mediterranean Sea over the last decades, in: Copernicus marine service ocean state report, issue 6, J. Oper. Oceanogr., 15, 49–56, 2022.
Desportes, C., Garric, G., Régnier, C., Drévillon, M., Parent, L., Drillet, Y., Masina, S., Storto, A., Mirouze, I., Cipollone, A., Zuo, H., Balmaseda, M., Peterson, D., Wood, R., Jackson, L., Mulet, S., Grenier, E., and Gounou, A.: EU Copernicus Marine Service Quality Information Document for the Global Ocean Ensemble Physics Reanalysis, GLOBAL_REANALYSIS_PHY_001_031, Issue 1.1, Mercator Ocean International, https://catalogue.marine.copernicus.eu/documents/QUID/CMEMS-GLO-QUID-001-031.pdf (last access: 3 May 2023), 2022.
Escudier, R., Clementi, E., Cipollone, A., Pistoia, J., Drudi, M., Grandi, A., Lyubartsev, V., Lecci, R., Aydogdu, A., Delrosso, D., Omar, M., Masina, S., Coppini, G., and Pinardi, N.: A High Resolution Reanalysis for the Mediterranean Sea, Front. Earth Sci., 9, 702285, https://doi.org/10.3389/feart.2021.702285, 2021.
Escudier, R., Clementi, E., Nigam, T., Aydogdu, A., Fini, E., Pistoia, J., Grandi, A., and Miraglio, P.: EU Copernicus Marine Service Quality Information Document for the Mediterranean Sea Physics Reanalysis, MEDSEA_MULTIYEAR_PHY_006_004, Issue 2.3, Mercator Ocean International, https://catalogue.marine.copernicus.eu/documents/QUID/CMEMS-MED-QUID-006-004.pdf (last access: 3 May 2023), 2022.
EU Copernicus Marine Service Product: Multi Observation Global Ocean 3D Temperature Salinity Height Geostrophic Current and MLD, Mercator Ocean International [data set], https://doi.org/10.48670/moi-00052, 2021.
EU Copernicus Marine Service Product: Mediterranean Sea Physics Reanalysis, Mercator Ocean International [data set], https://doi.org/10.25423/CMCC/MEDSEA_MULTIYEAR_PHY_006_004_E3R1, 2022a.
EU Copernicus Marine Service Product: Mediterranean Sea Physics Reanalysis INTERIM, Mercator Ocean International [data set], https://doi.org/10.25423/CMCC/MEDSEA_MULTIYEAR_PHY_006_004_E3R1I, 2022b.
EU Copernicus Marine Service Product: Global Ocean Ensemble Physics Reanalysis, Mercator Ocean International [data set], https://doi.org/10.48670/moi-00024, 2022c.
EU Copernicus Marine Service Product: Global Ocean-Delayed Mode gridded CORA – In-situ Observations objective analysis in Delayed Mode, INSITU_GLO_PHY_TS_OA_MY_013_052, Mercator Ocean International [data set], https://doi.org/10.17882/46219, 2022d.
Fedele, G., Mauri, E., Notarstefano, G., and Poulain, P. M.: Characterization of the Atlantic Water and Levantine Intermediate Water in the Mediterranean Sea using 20 years of Argo data, Ocean Sci., 18, 129–142, https://doi.org/10.5194/os-18-129-2022, 2022.
Gounou, A., Drevillon, M., and Clavier, M.: EU Copernicus Marine Service Product User Manual for the Global Ocean Ensemble Physics Reanalysis, GLOBAL_REANALYSIS_PHY_001_031, Issue 1.1, Mercator Ocean International, https://catalogue.marine.copernicus.eu/documents/PUM/CMEMS-GLO-PUM-001-031.pdf (last access: 3 May 2023), 2022.
Grenier, E., Verbrugge, N., Mulet, S., and Guinehut, S.: EU Copernicus Marine Service Quality Information Document for the Multi Observation Global Ocean 3D Temperature Salinity Height Geostrophic Current and MLD, MULTIOBS_GLO_PHY_TSUV_3D_MYNRT_015_012, Issue 1.1, Mercator Ocean International, https://catalogue.marine.copernicus.eu/documents/QUID/CMEMS-MOB-QUID-015-012.pdf (last access: 3 May 2023), 2021.
Guinehut, S.: EU Copernicus Marine Service Product User Manual for the Multi Observation Global Ocean 3D Temperature Salinity Height Geostrophic Current and MLD, MULTIOBS_GLO_PHY_TSUV_3D_MYNRT_015_012, Issue 1.1, Mercator Ocean International, https://catalogue.marine.copernicus.eu/documents/PUM/CMEMS-MOB-PUM-015-012.pdf (last access: 3 May 2023), 2021.
Guinehut, S., Dhomps, A.-L., Larnicol, G., and Le Traon, P.-Y.: High resolution 3-D temperature and salinity fields derived from in situ and satellite observations, Ocean Sci., 8, 845–857, https://doi.org/10.5194/os-8-845-2012, 2012.
Holliday, N. P., Bersch, M., Berx, B., Chafik, L., Cunningham, S., Florindo-López, C., Hátún, H., Johns, W., Josey, S. A., Larsen, K. M., and Mulet, S.: Ocean circulation causes the largest freshening event for 120 years in eastern subpolar North Atlantic, Nat. Commun., 11, 585, https://doi.org/10.1038/s41467-020-14474-y, 2020.
Jarosz, E., Teague, W. J., Book, J. W., and Beşiktepe, Ş. T.: Observed volume fluxes and mixing in the Dardanelles Strait, J. Geophys. Res.-Oceans, 118, 5007–5021, 2013.
Johnson, G. C., Hosoda, S., Jayne, S. R., Oke, P. R., Riser, S. C., Roemmich, D., Suga, T., Thierry, V., Wijffels, S. E., and Xu, J.: Argo-two decades: global oceanography, revolutionized, Annu. Rev. Mar. Sci., 14, 379–403, 2022.
Jordà, G., Von Schuckmann, K., Josey, S. A., Caniaux, G., García-Lafuente, J., Sammartino, S., Özsoy E., Polcher J., Notarstefano, G., Poulain, P. M., Adloff, F., Salat, J., Naranjo, C., Schroeder, K., Chiggiato, J., Sannino, G., and Macías, D.: The Mediterranean Sea heat and mass budgets: Estimates, uncertainties and perspectives, Prog. Oceanogr., 156, 174–208, https://doi.org/10.1016/j.pocean.2017.07.001, 2017.
Juza, M., Fernández-Mora, À., and Tintoré, J.: Sub-Regional Marine Heat Waves in the Mediterranean Sea From Observations: Long-Term Surface Changes, Sub-Surface and Coastal Responses, Front. Mar. Sci., 9, 785771, https://doi.org/10.3389/fmars.2022.785771, 2022.
Lecci, R., Drudi, M., Grandi, A., Cretì, S., and Clementi, E.: EU Copernicus Marine Service Product User Manual for the Mediterranean Sea Physics Reanalysis, MEDSEA_MULTIYEAR_PHY_006_004, Issue 2.3, Mercator Ocean International, https://catalogue.marine.copernicus.eu/documents/PUM/CMEMS-MED-PUM-006-004.pdf (last access: 3 May 2023), 2022.
Lellouche, J.-M., Le Galloudec, O., Drévillon, M., Régnier, C., Greiner, E., Garric, G., Ferry, N., Desportes, C., Testut, C.-E., Bricaud, C., Bourdallé-Badie, R., Tranchant, B., Benkiran, M., Drillet, Y., Daudin, A., and De Nicola, C.: Evaluation of global monitoring and forecasting systems at Mercator Océan, Ocean Sci., 9, 57–81, https://doi.org/10.5194/os-9-57-2013, 2013.
MacLachlan, C., Arribas, A., Peterson, K. A., Maidens, A., Fereday, D., Scaife, A. A., Gordon, M., Vellinga, M., Williams, A., Comer, R. E., Camp, J., Xavier, P., and Madec, G.: Global seasonal forecast system version 5 (GloSea5): A high-resolution seasonal forecast system, Q. J. Roy. Meteorol. Soc., 141, 1072–1084, https://doi.org/10.1002/qj.2396, 2014.
Masina, S. and Storto, A.: Reconstructing the recent past ocean variability: status and perspective, J. Mar. Res., 75, 727–764, 2017.
Pisano, A., Marullo, S., Artale, V., Falcini, F., Yang, C., Leonelli, F. E., Santoleri, R., and Buongiorno Nardelli, B.: New Evidence of Mediterranean Climate Change and Variability from Sea Surface Temperature Observations, Remote Sens., 12, 132, https://doi.org/10.3390/rs12010132, 2020.
Sannino, G., Sözer, A., and Özsoy, E.: A high-resolution modelling study of the Turkish Straits System, Ocean Dynam., 67, 397–432, 2017.
Schauer, U. and Losch, M.: “Freshwater” in the ocean is not a useful parameter in climate research, J. Phys. Oceanogr., 49, 2309–2321, 2019.
Schroeder, K., Chiggiato, J., Bryden, H. L., Borghini, M., and Ben Ismail, S.: Abrupt climate shift in the Western Mediterranean Sea, Scientific reports, 6, 1–7, 2016.
Skliris, N., Zika, J. D., Herold, L., Josey, S. A., and Marsh, R.: Mediterranean sea water budget long-term trend inferred from salinity observations, Clim. Dynam., 51, 2857–2876, https://doi.org/10.1007/s00382-017-4053-7, 2018.
Soto-Navarro, J., Criado-Aldeanueva, F., García-Lafuente, J., and Sánchez-Román, A.: Estimation of the Atlantic inflow through the Strait of Gibraltar from climatological and in situ data, J. Geophys. Res.-Oceans, 115, C10023, https://doi.org/10.1029/2010JC006302, 2010.
Storto, A., Masina, S., and Navarra, A.: Evaluation of the CMCC eddy-permitting global ocean physical reanalysis system (C-GLORS, 1982–2012) and its assimilation components, Q. J. R. Meteorol. Soc., 142, 738–758, https://doi.org/10.1002/qj.2673, 2016.
Storto, A., Masina, S., Simoncelli, S., Iovino, D., Cipollone, A., Drevillon, M., Drillet, Y., von Schuckman, K., Parent, L., Garric, G., and Greiner, E.: The added value of the multi-system spread information for ocean heat content and steric sea level investigations in the CMEMS GREP ensemble reanalysis product, Clim. Dynam., 53, 287–312, https://doi.org/10.1007/s00382-018-4585-5, 2019a.
Storto, A., Alvera-Azcárate, A., Balmaseda, M. A., Barth, A., Chevallier, M., Counillon, F., Domingues, C. M., Drevillon, M., Drillet, Y., Forget, G., Garric, G., Haines, K., Hernandez, F., Iovino, D., Jackson, L. C., Lellouche, J.-M., Masina, S., Mayer, M., Oke, P. R., Penny, S. G., Peterson, K. A., Yang, C., and Zuo, H.: Ocean reanalyses: recent advances and unsolved challenges, Front. Mar. Sci., 6, 418, https://doi.org/10.3389/fmars.2019.00418, 2019b.
Szekely, T.: EU Copernicus Marine Service Quality Information Document for the Global Ocean-Delayed Mode gridded CORA – In-situ Observations objective analysis in Delayed Mode, INSITU_GLO_PHY_TS_OA_MY_013_052, issue 1.2, Mercator Ocean International, https://catalogue.marine.copernicus.eu/documents/QUID/CMEMS-INS-QUID-013-052.pdf (last access: 4 April 2023), 2022a.
Szekely, T.: EU Copernicus Marine Service Product User Manual for the Global Ocean-Delayed Mode gridded CORA – In-situ Observations objective analysis in Delayed Mode, INSITU_GLO_PHY_TS_OA_MY_013_052, issue 1.10, Mercator Ocean International, https://catalogue.marine.copernicus.eu/documents/PUM/CMEMS-INS-PUM-013-002-052.pdf (last access: 4 April 2023), 2022b.
Szekely, T., Gourrion, J., Pouliquen, S., and Reverdin, G.: The CORA 5.2 dataset for global in situ temperature and salinity measurements: data description and validation, Ocean Sci., 15, 1601–1614, https://doi.org/10.5194/os-15-1601-2019, 2019.
Tuel, A. and Eltahir, E. A. B.: Why Is the Mediterranean a Climate Change Hot Spot?, J. Climate, 33, 5829–5843, https://journals.ametsoc.org/view/journals/clim/33/14/JCLI-D-19-0910.1.xml (retrieved 25 Jannuary 2022), 2020.
Zunino, P., Schroeder, K., Vargas-Yáñez, M., Gasparini, G. P., Coppola, L., García-Martínez, M. C., and Moya-Ruiz, F.: Effects of the Western Mediterranean Transition on the resident water masses: Pure warming, pure freshening and pure heaving, J. Mar. Syst., 15, 96–97, https://doi.org/10.1016/j.jmarsys.2012.01.011, 2012.
Zuo, H., Balmaseda, M. A., and Mogensen, K.: The new eddy-permitting ORAP5 ocean reanalysis: description, evaluation and uncertainties in climate signals, Clim. Dynam., 49, 791–811, https://doi.org/10.1007/s00382-015-2675-1, 2017.