the Creative Commons Attribution 4.0 License.
the Creative Commons Attribution 4.0 License.
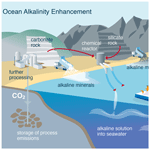
Climate targets, carbon dioxide removal, and the potential role of ocean alkalinity enhancement
Lennart T. Bach
Rosalind E. M. Rickaby
Terre Satterfield
Romany Webb
Jean-Pierre Gattuso
The Paris Agreement to limit global warming to well below 2 ∘C requires the ambitious reduction in greenhouse gas emissions and the balancing of remaining emissions through carbon sinks (i.e., the deployment of carbon dioxide removal or CDR). While ambitious climate mitigation scenarios until now primarily consider land-based CDR methods, there is growing concern about their potential to deliver sufficient CDR, and marine CDR options are receiving more and more interest. Based on idealized theoretical studies, ocean alkalinity enhancement (OAE) appears as a promising marine CDR method. However, the knowledge base is insufficient for a robust assessment of its practical feasibility, of its side effects, social and governance aspects, and monitoring and verification issues. A number of research efforts aims to improve this in a timely manner. We provide an overview on the current situation of developing OAE as a marine CDR method and describe the history that has led to the creation of the OAE research best practices guide.
- Article
(2020 KB) - Full-text XML
- BibTeX
- EndNote
A key finding of climate research in recent decades is that the increase in global mean surface air temperature since the beginning of industrialization is proportional to cumulative emissions of carbon dioxide (CO2), which is the major anthropogenic greenhouse gas (Matthews et al., 2009). The Paris Agreement's goal of limiting global warming to well below 2 ∘C, and ideally 1.5 ∘C above preindustrial levels (UNFCCC, 2015), can thus be converted to a remaining carbon budget that, for current global emissions, will be used up in a few years for the 1.5 ∘C target and about 2 decades for the 2 ∘C target (United Nations Environmental Programme, 2022). The Paris Agreement thus explicitly demands ambitious reductions in anthropogenic greenhouse gas emissions and the balancing of hard-to-abate emissions through carbon sinks in the second-half of the 21st century (UNFCCC, 2015). The balance to be achieved is also called net zero and is a qualitatively new element compared to previous climate protection agreements.
Arresting global warming will require net-zero CO2 emissions. Non-CO2 greenhouse gases (GHGs), in particular nitrous oxide and methane, also contribute to current warming. However, because their lifetime in the atmosphere is considerably shorter than that of CO2, arresting global warming does not require net-zero emissions for non-CO2 GHGs (Allen et al., 2022). Nevertheless, increases in non-CO2 GHG emissions may lead to further temperature rise, whereas a decrease in non-CO2 GHG emissions will relatively quickly reduce atmospheric concentrations of the respective non-CO2 GHG and thus radiative forcing and global warming. In order to achieve the long-term temperature goal, parties to the Paris Agreement agreed to reach global peaking of GHG emissions as soon as possible, to undertake rapid reductions thereafter, and to achieve a balance, i.e., net zero, between anthropogenic emissions by sources and removals by sinks of greenhouse gases in the second-half of this century. The Paris Agreement adopts the United Nations Framework Convention on Climate Change (UNFCCC) definition of “sink”, which refers to “any process, activity or mechanism which removes a greenhouse gas … from the atmosphere” and thus encompasses both ecosystem-based and more technological or engineered removal approaches. Presently, no viable method exists for large-scale removal of non-CO2 GHGs. Therefore, carbon dioxide removal will likely have to balance not only hard-to-abate residual emissions of CO2, e.g., from cement production, waste incineration, aviation, and maritime transport, but also emissions of non-CO2 GHGs, in particular from agriculture.
The amount of these residual emissions needs to be politically and socially viable. In principle, all hard-to-abate emissions are technically avoidable, e.g., by switching from fossil to renewable energy, by capturing and safely storing CO2 from process emissions (e.g., cement production using renewable energy), or by avoiding the processes that lead to emissions. Particularly in the agricultural sector, avoiding all emissions appears impossible without critical societal impacts; rice production and the raising of livestock are associated with methane production, and any use of nitrogen fertilizer is associated with nitrous oxide production, and both of these are potent non-CO2 greenhouse gases. The exact amount of residual emissions is thus largely an issue of economic and social costs and society's ambition to avoid emissions. Which emissions are deemed unavoidable also vary across historical and political contexts and are influenced by claims as to what is regarded as legitimately possible (Lund et al., 2023). Ultimately, decisions about the amount of residual emissions depend on values, norms, and interests. Current scenarios assume that, by mid-century, residual emissions will amount to between 10 % and 20 % of today's emissions (i.e., about 6 to 12 Gt CO2e yr−1 globally), where CO2e includes the CO2 equivalents of non-CO2 GHGs that are estimated to contribute half to two-thirds of the residual emissions (Buck et al., 2023).
Current global carbon dioxide removal (CDR) has been estimated to be near 2 Gt CO2 yr−1 almost exclusively by the conventional management of land, primarily forest management (Grassi et al., 2021), afforestation, and reforestation and with only 0.002 Gt CO2 yr−1 by novel CDR schemes comprising bioenergy with carbon capture and storage (BECCS), direct air capture with carbon storage (DACCS), enhanced weathering, and marine CDR, also sometime called ocean CDR, including ocean alkalinity enhancement (OAE) as a subcategory (Smith et al., 2023). According to the State of Carbon Dioxide Removal report (Smith et al., 2023), the deployment of novel CDR approaches will have to increase by 3 orders of magnitude by mid-century in order to reach net-zero emissions, even in the most ambitious emission reduction scenarios. Note that many scenarios used in the recent Intergovernmental Panel on Climate Change Sixth Assessment Report (IPCC, 2022) assume that emissions turn net negative after having reached net zero (Fig. 1), which would allow a net cooling and is also deemed necessary for so-called temperature overshoot scenarios (Geden and Löschel, 2017) that receive more attention the longer it takes to drastically reduce emissions. It is, however, currently unclear how to best incentivize and govern net negative emissions.
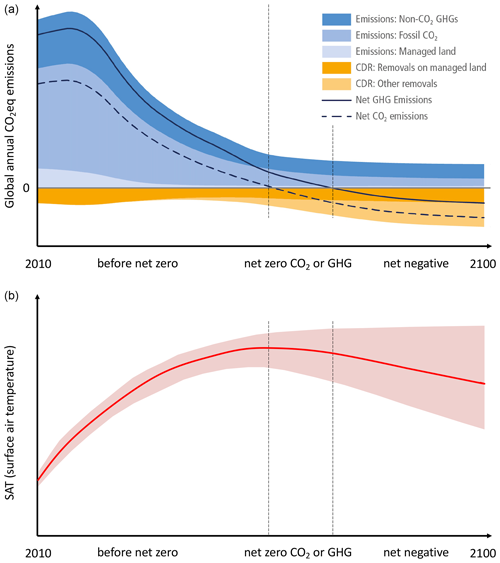
Figure 1(a) The role of CO2 removal (CDR) in a stylized pathway of ambitious climate. Dark orange illustrates the CO2 removals from land management, and light orange illustrates removal from other CDR methods, including ocean-based methods. Note that net-zero CO2 is reached well before net-zero greenhouse gas (GHG), and the amount of CDR required for net-zero CO2 can be substantially smaller than the amount of CDR required for non-zero GHG. Any contribution of ocean alkalinity enhancement would be included in light orange (CDR: other removals). This panel (a) has been modified from IPCC (2022, Cross-Chapter Box 8, Fig. 2). (b) The corresponding global surface air temperature, with shading indicating a typical uncertainty range.
While the current climate goal of the UNFCCC is to limit the temperature rise to 2 or 1.5 ∘C relative to preindustrial levels, one could also envisage climate targets that aim to reduce global temperatures further toward preindustrial levels – and much faster than the tens to hundreds of millennia that planetary feedbacks would take to do so (Archer et al., 2009). Should humanity aim for a faster restoration of the planetary thermal balance to preindustrial times, then CDR would be a prime mechanism, with the deployment required being well beyond the current net-zero targets.
Traditionally, the focus of CDR has been on land-based methods such as reforestation and afforestation or BECCS. While these approaches certainly have some potential, there are unresolved issues related to land use competition and associated political and societal feasibility challenges, and it is currently unclear if and how their combined deployment will be possible at scales sufficient to meet the net-zero target by mid-century (The Land Gap Report, Dooley et al., 2022). It is thus unlikely that such terrestrial-ecosystem-based solutions alone will be sufficient to achieve net zero (Smith et al., 2023), and therefore, novel approaches will also have to be applied to a considerable extent. None of these is ready for large-scale deployment today. Transparent research into the efficacy, risks, and benefits of different approaches is urgently needed, and the societal debate on what counts as residual emissions and whether and how to deploy different CDR approaches must begin quickly so that appropriate processes can be developed in time, well-informed decisions can be made about research, development, and deployment, and mechanisms can be devised to regulate such use responsibly. Importantly, deployment at a scale of hundreds to thousands of megatons of CO2 per year could compete with other societal demands for land, water, and energy (Lawrence et al., 2018). Marine CDR has the potential to reduce the need for land and freshwater resources. Large-scale marine CDR approaches, however, may struggle to achieve public acceptance (Bertram and Merk, 2020; Nawaz et al., 2023).
Marine CDR options are receiving more and more interest, acknowledging that the ocean has already absorbed more than a quarter of the anthropogenic CO2 emissions and would, on timescales of thousands to hundreds of thousands of years, take up most of the remaining emissions (Archer and Brovkin, 2008), as it has done with natural high-CO2 excursions in the Earth's geological past. The ocean holds more than 50 times as much carbon (primarily in the form of dissolved inorganic carbon) as the preindustrial atmosphere and about 20 times as much as the carbon stored in global terrestrial plants and soils (Carlson et al., 2001). Its theoretical carbon storage potential appears large when compared to the atmospheric and terrestrial carbon pools. However, increasing the oceanic carbon pool will affect the marine environment and may put additional pressure on marine ecosystems. The current level of scientific understanding of marine CDR is low, and more research is required to comprehensively assess the diverse portfolio of proposed options (e.g., NASEM, 2021). A particular challenge for marine CDR concerns the monitoring and verification of any CDR-induced carbon fluxes and carbon storage, which is essential for reliable and honest carbon crediting (Boyd et al., 2023). The detection and attribution of CDR signals is particularly difficult, due to the large natural marine carbon pool that already contains a considerable anthropogenic signal. The high temporal and spatial variability in these signals, as well as the temporal and spatial decoupling of air–sea CO2 fluxes and carbon storage in the interior ocean, pose specific challenges to the detection and attribution of CDR. The determination of a baseline, the additional carbon sequestered beyond the baseline, and the quantification of carbon storage durability will likely be associated with considerable uncertainties. A key aspect of monitoring, reporting, and verification (MRV) is the development of transparent schemes that allow a reliable determination of CDR and of consequent impacts on the carbon cycle, and hence climate, as well as the association of carbon credits with individual CDR activities.
Currently considered marine CDR approaches include (1) biological methods, such as photosynthetic carbon fixation by microalgae, macrophytes (e.g., seaweeds), or mangrove trees and the subsequent storage of carbon in the deep ocean or in coastal sediments; and (2) abiotic methods that aim to alter the carbonate chemistry of seawater in a way that enhances the air–sea flux of CO2 and subsequently stores atmospheric carbon as dissolved inorganic carbon in seawater. Hybrid biological, physical, and/or chemical marine CDR approaches are also considered (artificial upwelling or downwelling, marine BECCS, bio-enhanced alkalinity generation, hybrid ocean–geochemical approaches, etc.). Among marine CDR methods investigated, abiotic approaches have been assessed as being those with the lowest knowledge base and highest efficacy (Gattuso et al., 2018; NASEM, 2021). Improving their knowledge base therefore appears critical, and we focus here on the ocean alkalinity enhancement.
Ocean alkalinity enhancement is a marine CDR concept with high theoretical sequestration potential in the range of 3 to 30 Gt CO2 yr−1 (Köhler et al., 2013; Renforth and Henderson, 2017; Feng et al., 2017), for which a number of technical deployment approaches has been suggested (Fig. 2). Alkalinity, which is the excess of proton acceptors over donors, is a chemical concept that largely determines the storage capacity for CO2 in seawater. OAE aims to enhance alkalinity by adding alkaline material to the surface ocean or by removing acid from seawater via electrochemistry. Alkalinity enhancement results in the consumption of protons, which is a corresponding increase in the pH, results in a decrease in the partial pressure of CO2 in seawater. If applied to the surface ocean, and depending on the initial air–sea CO2 gradient, it would promote CO2 uptake from – or lessen CO2 release to – the atmosphere, in both cases leading to a net reduction in atmospheric CO2 at the expense of an increase in the oceanic pool of dissolved inorganic carbon. The atmospheric CO2 absorbed via OAE-induced air–sea gas exchange is essentially stored in the form of dissolved bicarbonate and carbonate ions that do not exchange with the atmosphere.
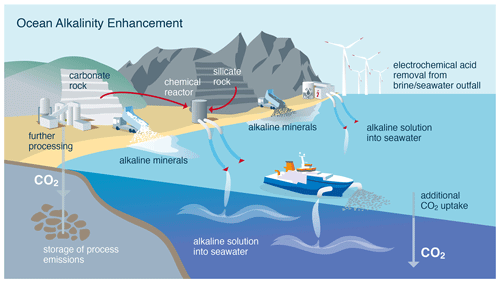
Figure 2Illustration of the various methods that have been proposed as ocean alkalinity enhancement measures to achieve carbon dioxide removal.
When applied to the surface ocean, OAE can rely on air–sea gas exchange to at least partially restore the OAE-induced decrease in the partial pressure of CO2. Air–sea gas equilibration of CO2 can take months to years (Jones et al., 2014) and may pose specific challenges to MRV (He and Tyka, 2023). However, along the path to equilibration, air–sea CO2 fluxes approach zero and would, for otherwise constant environmental conditions, follow an inverse exponential function for which a disproportionate share of the total CO2 flux occurs at the beginning of the equilibration period. The complex impacts of mixing and transport of water masses in reality make direct observations of the CO2 influx unfeasible. Numerical models may be required for reliable quantification of air–sea gas exchange, but their skill has yet to be demonstrated (Bach et al., 2023). OAE can also be applied by adding alkalinity to chemical reactors upstream that could at least partially pre-equilibrate the alkalized seawater with additional CO2 taken either from ambient air or from CO2 waste streams. If CO2 is taken from waste streams, then this would, technically, correspond to emissions avoidance and not CDR. Also, if this CO2 were taken from ambient air via, e.g., direct air capture facilities or bioenergy plants, then CDR would be termed according to the process that removes additional CO2 from the atmosphere and not the process that provides terminal carbon storage. OAE applied to chemical reactors or to the surface ocean qualifies as marine CDR if it leads to a net removal of CO2 from the atmosphere, either by increasing the flux of CO2 from the atmosphere to the ocean or by reducing the emissions of CO2 from the ocean to the atmosphere. Hybrid schemes that combine emission reduction by dissolving minerals with acidic CO2 waste streams in chemical reactors to generate dissolved alkaline solutions to be added into the ocean for subsequent marine CDR can also be envisaged.
OAE was positioned in the “concept stage” cluster of a recent assessment of ocean-based measures for climate action (Gattuso et al., 2021). This cluster was defined for measures with potentially very high effectiveness but with feasibility and cost-effectiveness levels which have yet to be demonstrated. The assessment highlighted the urgent need to improve knowledge on concept stage measures because the full implementation of proven measures runs the risk of falling short of providing enough of a cost-effective CDR capacity. The attractive aspects of OAE compared to many other methods, in particular those that store carbon in biomass, are its potential to reduce ocean acidification at least locally (Albright et al., 2016) and the theoretical durability of storage over several tens to hundreds of thousands of years. An effective leakage of CO2, either via enhanced CO2 flux back to the atmosphere or by reduced CO2 uptake from the atmosphere compared to a baseline scenario, can result from enhanced formation and reduced dissolution of carbonate minerals in the water column or at the sea floor. Possible leakage effects via the impacts of OAE on pelagic calcifiers are uncertain (Bach et al., 2019), and feedbacks via changes in the dissolution and preservation of carbonates on the sea floor operate on timescales of hundreds to thousands of years (e.g., Gehlen et al., 2008). While there is little indication that leakage is a major concern for OAE on shorter than centennial timescales, a quantitative assessment of leakage across the spectrum of timescales is lacking. Frequently mentioned drawbacks of OAE are (i) the amount and the quality of alkaline material that is needed (whether mined in the case of mineral-based approaches or generated from waste brine in electrochemical approaches) and the energy required (whether mining, grinding, and transport for mineral-based approaches or the source of electricity for electrochemical approaches); and (ii) the difficulty of reliably quantifying CDR (MRV). Regarding point (i), all known CDR methods require, at climate-relevant scales, the movement of large amounts of matter. In addition to abundant carbonate and silicate minerals, a number of industrial waste products or artificial minerals can also be considered to be alkalinity sources (Renforth, 2019; Caserini et al., 2022). Employing these for OAE would require proper accounting for any CO2 emitted in their production (e.g., Ca(OH)2 or Mg(OH)2 produced through the calcination of CaCO3 or MgCO3, respectively). Overall, there is no shortage of alkaline materials on the planet (Caserini et al., 2022). Regarding point (ii), MRV is indeed a challenge and is addressed by Ho et al. (2023, this Guide).
So far, the CDR potential of OAE has essentially been inferred from modeling and techno-economic studies (Kheshgi, 1995; Harvey, 2008), including spatially resolved global or regional models (e.g., Ilyina et al., 2013; Keller et al., 2014; Hauck et al., 2016; Wang et al., 2023). Such models employ simplified representations of marine biogeochemistry, rudimentary descriptions of marine ecosystems, and typically simulate OAE as being the addition, often instantaneously, of pure alkalinity or of olivine minerals consisting of silicate, iron, and alkalinity. Such studies can provide large-scale estimates of the theoretical CDR potential of OAE. Small-scale experimental studies can complement this with insight into realizable effectiveness of alkalinity addition and with the investigation of impacts that cannot be predicted from simplified modeled systems, such as environmental side-effects. The first experimental studies have started only recently and have already generated novel insight into issues regarding the actual delivery of alkalinity, in particular the risk of calcium carbonate precipitation that may negate intended CDR effects (Fuhr et al., 2022; Moras et al., 2022; Hartmann et al., 2023), and ecological impacts (Ferderer et al., 2022), and further research efforts are underway. Some information on the biogeochemical and ecological impacts of OAE might be gained from experimental work on ocean acidification that has been carried out during recent decades. Indeed, a first OAE field experiment was carried out in the context of ocean acidification research. It used alkalinity addition to demonstrate that ocean acidification is detrimental to coral reef calcification and that alkalinity addition can alleviate some effects of ocean acidification (Albright et al., 2016). Insight into the possible impacts of OAE on marine organisms can be gained from research by the shellfish industry investigating the utility of so-called sweetening of the water through addition of mainly soda ash (Na2CO3), a practice utilized in shellfish hatcheries for decades, and also in the academic and industrial fields of river liming, which dissolved primarily CaCO3 and dolomite in higher-latitude watersheds to offset the effects of acid rain in the 1960s and 1970s but is still practiced today in Canada and some Scandinavian countries, among other places (Mant et al., 2013).
Still, crucial knowledge gaps exist. Issues to be researched include the method of alkalinity addition, the alkaline materials to be used, and their processing, the key attributes of ideal locations for deployment, the CDR potential that can be realized on given timescales, the durability of the carbon storage, biogeochemical, and ecological co-benefits and risks, as well as MRV and the economic, legal, social, and ethical aspects of OAE. Of particular relevance for OAE and most other marine CDR methods is the regulatory perspective at international level. This is required to govern activities affecting the ocean as part of the global commons.
The very few (fewer than 10, according to the authors' knowledge) field trials that have been carried out so far, or are being discussed in the year 2023, have the potential to take up a few tonnes of CO2 per trial. For the various OAE approaches, technology-readiness levels (TRLs) are relatively low and are generally rated as being 1–2 by Smith et al. (2023), 3–4 for specific approaches (Foteinis et al., 2022), and possibly approaching 5–6 for methods with the first field trials in preparation or underway (see Eisaman et al., 2023, this Guide). Scaling up the CO2 uptake by several orders of magnitude to many millions of tonnes per year or possibly even a billions of tonnes per year by mid-century is extremely ambitious. It would require all instruments, measures, and policies put in place that can advance every option forward from its current readiness level. In their State of Carbon Dioxide Removal report, Smith et al. (2023) estimated that so-called novel CDR methods, which include OAE, would need to be scaled up about by a factor 30 by 2030 and a factor of 1300 by mid-century in order to meet the demand expected for reaching promised climate goals. Required average annual OAE growth rates will have to be around 50 %, which is extremely ambitious compared to, for example, an average of 9 % annual increase in the global capacity of renewable energy (IRENA, 2021). Whether or not CDR and OAE specifically can be scaled up sufficiently by mid-century will depend on progress over the next decade, which Smith et al. (2023) call the “novel CDR's formative years”. A possible advantage of most OAE methods is that, technologically, they appear relatively simple and rely, to a substantial degree, on technology that exists already for processing different mineral resources at annual rates similar to those that may be required by OAE by mid-century. A possible roadblock for rapidly scaling up OAE may be a lack of public acceptance (Bertram and Merk, 2020; Nawaz et al., 2023).
In addition to technological challenges and acceptability issues that would need to be resolved, appropriate governance schemes will be needed if OAE is to be deployed at climatically relevant scales (GESAMP, 2019; Boettcher et al., 2021). The 2013 amendment to the London Protocol offers an approach for governing marine CDR, with a focus on ocean fertilization, but would need to be developed further with regards to OAE (see Steenkamp and Webb, 2023, this Guide). Interactions between OAE and other ocean-based activities will also need to be considered (e.g., via marine spatial planning), and any climate-relevant OAE deployment would require new or significantly expanded climate policies and financing schemes. The inclusion of OAE in carbon markets will require the establishment of robust MRV procedures.
All of these issues need to be resolved before OAE can be implemented at a large scale. Achieving this by mid-century is challenging but not impossible. Research is urgently required on all aspects that are addressed in the various papers of this volume.
Given the urgency of establishing a portfolio of CDR options, a rapid improvement of our understanding of the carbon storage potential and of the co-benefits and risks of OAE is needed. This requires responsible, efficient, and transparent scientific research in order to generate new and reliable information, allowing for rapid sharing, testing, and synthesis of the results. With the first publicly funded research projects having started in several countries, philanthropy funding a number of research projects to accelerate the scientific progress, and start-ups working on enhancing technological readiness and developing scalable methodologies, this has motivated us to develop a best practice guide for OAE research.
The papers included in this guide describe current knowledge on the strengths and weaknesses of different OAE approaches, scientific uncertainties, biological and ecological impacts, knowledge gaps, and research needs. Recommendations for the experimental setup of a laboratory, pelagic and benthic mesocosms, and field experiments, as well as for modeling approaches, are provided. The guide also discusses the legal context in which research occurs and offers recommendations for responsible research and innovation, public engagement, data reporting and sharing, MRV, and attribution.
The best practice guide aims at fostering intercomparison and synthesis efforts of different studies evaluating the potential, effectiveness, and durability of OAE. This will help to improve knowledge sharing and information gain and thereby speed up scientific progress at a time when robust information about OAE as a carbon dioxide removal option is urgently needed to enable society to define and design appropriate actions to reach agreed climate goals.
This research field is in its infancy and is rapidly evolving. The broader legal and social contexts in which research occur are also undergoing change. What we designate as best practice in this guide today may not be considered the best practice in the future. As such, our guide comprises our current understanding of OAE, but it is critical that users remain up to date with respect to the literature published after publication of the OAE guide. There will almost certainly be improvements in protocols as the field develops, and everyone is invited to contribute to this process.
Best practice guides have proven useful when new areas of research open up, often bringing together scientists from different fields and with different methodological backgrounds. One example is the Guide to best practices in ocean acidification research and data reporting (Riebesell et al., 2010), in which the project lead, Jean-Pierre Gattuso, the scientific coordinator, Andreas Oschlies, and a number of authors of this guide were involved. The ocean acidification guide had an enormous catalytic effect in growing the field of ocean acidification research by lowering the barrier to entry and making the comparison of different studies and the generation of synthesis products more straightforward. The expectation is that the present guide on OAE research will have a similar impact on the OAE community and ocean CDR field at large and also provide guidelines for ensuring that OAE research is conducted responsibly and most efficiently for the public good.
In summer 2022, Jean-Pierre Gattuso and Andreas Oschlies sent a proposal to the ClimateWorks Foundation with a request for funding to produce a detailed guide that outlines all the relevant approaches available for researching ocean alkalinity enhancement as a carbon dioxide removal method. The requested funding for a part-time project manager, a 3 d in-person workshop of the chapter lead authors, and costs for the production, publication, and printing of the guide (a total sum of USD 170 000) was approved. A steering committee, consisting of the authors of the present paper, was established and had several online meetings to develop outline and a conflict-of-interest form that all authors would have to sign in order to ensure transparency, best scientific knowledge, and the absence of conflicts of interest. Lead authors for each paper of the guide were chosen by the steering committee based on experience, scholarship, and diversity. In consultation with the steering committee, all lead authors then chose the co-leads and additional authors of their respective papers.
In early 2023, a 3 d in-person workshop of the steering committee and lead authors took place in Villefranche-sur-Mer, France. All paper outlines were discussed, gaps identified, and the timeline agreed upon. The lead authors were responsible for developing their papers, with support from the scientific project manager. A public website (https://oae-best-practice.carbondioxide-removal.eu, last access: 26 October 2023) with a list of papers and lead authors was set up and advertised via social media and the CDR news stream (https://www.carbondioxide-removal.eu, last access: 26 October 2023). An internal review was initiated in May 2023. All papers were submitted to State of the Planet in order to allow for public review and to ensure that the OAE Guide 23 provides state-of-the-art information.
Key recommendations
-
Research on ocean alkalinity enhancement should consider and, whenever appropriate, follow the best practices lined out in the OAE Guide 23.
-
Results of all experiments should be shared transparently, irrespective of whether experimental outcomes are considered positive (e.g., affirmative of the experimenters' prior assumptions), negative, or neutral. This includes full transparency with respect to OAE research that provides additional complications and/or roadblocks to OAE implementation.
-
We recommend establishing a public registry for OAE field experiments, where all field experiments should be registered before the experiment is carried out.
-
Researchers on OAE should help to further develop and improve the best practices outlined here and eventually strive for an update of the OAE Guide 23 in the future.
No data sets were used in this article.
AO conceptualized the paper project. All authors contributed to the writing of the initial draft, the responses to the reviewers, and the revision.
Competing interests are declared in a summary for the entire volume at: https://sp.copernicus.org/articles/sp-oae2023-ci-summary.zip.
Publisher’s note: Copernicus Publications remains neutral with regard to jurisdictional claims made in the text, published maps, institutional affiliations, or any other geographical representation in this paper. While Copernicus Publications makes every effort to include appropriate place names, the final responsibility lies with the authors.
We thank the Ocean Acidification and other ocean Changes – Impacts and Solutions (OACIS), an initiative of the Prince Albert II of Monaco Foundation, for its support throughout the project. We extend our gratitude to the Villefranche Oceanographic Laboratory for supporting the meeting of the lead authors in January 2023. We also thank Judith Meyer and Angela Stevenson for outstanding management of the production of the OAE Guide 23. We thank Miranda Boettcher, Kai Schulz, Matt Eisaman, and Greg Rau for constructive comments on earlier versions of this work. Andreas Oschlies acknowledges funding from the European Union's Horizon 2020 research and innovation program (grant no. 869357; project OceanNETs: Ocean-based Negative Emission Technologies analyzing the feasibility, risks, and co-benefits of ocean-based negative emission technologies for stabilizing the climate) and from the German Federal Ministry of Education and Research (grant no. 03F0895; project RETAKE, DAM Mission “Marine carbon sinks in decarbonization pathways”; CDRmare). We are grateful to Justin Ries and three anonymous referees for providing constructive comments that helped to improve the paper.
This research has been supported by the ClimateWorks Foundation (grant no. 22-0296), the Prince Albert II of Monaco Foundation. It has also been supported by the European Union's Horizon 2020 research and innovation program (grant no. 869357; project OceanNETs: Ocean-based Negative Emission Technologies analyzing the feasibility, risks, and co-benefits of ocean-based negative emission technologies for stabilizing the climate) and the German Federal Ministry of Education and Research (grant no. 03F0895; project RETAKE, DAM Mission “Marine carbon sinks in decarbonization pathways”; CDRmare).
The article processing charges for this open-access publication were covered by the GEOMAR Helmholtz Centre for Ocean Research Kiel.
This paper was edited by Katja Fennel and reviewed by Justin Ries and three anonymous referees.
Albright, R., Caldeira, L., Hosfelt, J., Kwiatkowski, L., Maclaren, J. K., Mason, B. M., Nebuchina, Y., Ninokawa, A., Pongratz, J., Ricke, K. L., Rivlin, T., Schneider, K., Sesboue, M., Shamberger, K., Silverman, J., Wolfe, Zhu, K., and Caldeira, K.: Reversal of ocean acidification enhances net coral reef calcification, Nature, 531, 362–365, 2016.
Allen, M. R., Friedlingstein, P., Girardin, C. A. J., Jenkins, S., Malhi, Y., Mitchell-Larson, E., Peters, G. P., and Rajamani, L.: Net zero: Science, origins, and implications, Annu. Rev. Env. Resour., 47, 849–887, 2022.
Archer, D. and Brovkin, V.: The millennial atmospheric lifetime of anthropogenic CO2, Climatic Change, 90, 283–297, 2008.
Archer, D., Eby, M., Brovkin, V., Ridgwell, A., Cao, L., Mikolajewicz, U., Caldeira, K., Matsumoto, K., Munhoven, G., Montenegro, A., and Tokos, K.: Atmospheric lifetime of fossil fuel carbon dioxide, Annu. Rev. Earth Pl. Sc., 37, 117–134, 2009.
Bach, L. T., Gill, S. J., Rickaby, R. E. M., Gore, S., and Renforth, P.: CO2 removal with enhanced weathering and ocean alkalinity enhancement: Potential risks and co-benefits for marine pelagic ecosystems, Front. Climate, 1, 7, https://doi.org/10.3389/fclim.2019.00007, 2019.
Bach, L. T., Ho, D. T., Boyd, P. W., and Tyka, M. D.: Toward a consensus framework to evaluate air–sea CO2 equilibration for marine CO2 removal, Limnol. Oceanogr.-Lett., 8, 685–691, https://doi.org/10.1002/lol2.10330, 2023.
Bertram, C. and Merk, C.: Public perceptions of ocean-based carbon dioxide removal: The nature-engineering divide?, Front. Climate, 2, 594194, https://doi.org/10.3389/fclim.2020.594194, 2020.
Boettcher, M., Brent, K., Buck, H. J., Low, S., McLaren, D., and Mengis, N.: Navigating potential hype and opportunity in governing marine carbon removal, Front. Climate, 3, 664456, https://doi.org/10.3389/fclim.2021.664456, 2021.
Boyd, P. W., Bach, L. T., Holden, R., and Turney, C.: Carbon offsets aren't helping the planet – for ways to fix them, Nature, 620, 947–949, 2023.
Buck, H. J., Carton, W., Lund, J. F., and Markusson, N.: Why residual emissions matter right now, Nat. Clim. Change, 13, 351–358, 2023.
Carlson, C. A., Bates, N. R., Hansell, D. A., and Steinberg, D. K.: Carbon Cycle, In: Encyclopedia of Ocean Science, 2nd edn., edited by: Steele, J., Thorpe, S., and Turekian K., Academic Press, Oxford, 477–486, https://doi.org/10.1016/B978-012374473-9.00272-1, 2001.
Caserini, S., Storni, N., and Grosso, M.: The availability of limestone and other raw materials for ocean alkalinity enhancement, Global Biogeochem. Cy., 36, e2021GB007246, https://doi.org/10.1029/2021GB007246, 2022.
Dooley, K., Keith, H., Larson, A., Catacora-Vargas, G., Carton, W., Christiansen, K., Enokenwa Baa, O., Frechette, A., Hugh, S., Ivetic, N., Lim, L., Lund, J., Luqman, M., Mackey, B., Monterroso, I., Ojha, H., Perfecto, I., Riamit, K., Robiou du Pont, Y., and Young, V.: The Land Gap Report, https://www.landgap.org/ (last access: 26 October 2023), 2022.
Eisaman, M. D., Geilert, S., Renforth, P., Bastianini, L., Campbell, J., Dale, A. W., Foteinis, S., Grasse, P., Hawrot, O., Löscher, C. R., Rau, G. H., and Rønning, J.: Assessing the technical aspects of ocean-alkalinity-enhancement approaches, in: Guide to Best Practices in Ocean Alkalinity Enhancement Research, edited by: Oschlies, A., Stevenson, A., Bach, L. T., Fennel, K., Rickaby, R. E. M., Satterfield, T., Webb, R., and Gattuso, J.-P., Copernicus Publications, State Planet, 2-oae2023, 3, https://doi.org/10.5194/sp-2-oae2023-3-2023, 2023.
Feng, E., Y., Koeve, W., Keller, D. P., and Oschlies, A.: Model-based assessment of the CO2 sequestration potential of coastal ocean alkalinization, Earth's Future, 5, 1252–1266, https://doi.org/10.1002/2017EF000659, 2017.
Ferderer, A., Chase, Z., Kennedy, F., Schulz, K. G., and Bach, L. T.: Assessing the influence of ocean alkalinity enhancement on a coastal phytoplankton community, Biogeosciences, 19, 5375–5399, https://doi.org/10.5194/bg-19-5375-2022, 2022.
Foteinis, S., Andresen, J., Campo, F., Caserini, S., and Renforth, P.: Life cycle assessment of ocean liming for carbon dioxide removal from the atmosphere, J. Clean. Prod., 370, 133309, https://doi.org/10.1016/j.jclepro.2022.133309, 2022.
Fuhr, M., Geilert, S., Schmidt, M., Liebetrau, V., Vogt, C., Ledwig, B., and Wallmann, K.: Kinetics of olivine weathering in seawater: An experimental study, Front. Climate, 4, 831587, https://doi.org/10.3389/fclim.2022.831587, 2022.
Gattuso, J.-P., Magnan, A. K., Bopp, L., Cheung, W. W. L., Duarte, C. M., Hinkel, J., Mcleod, E., Micheli, F., Oschlies, A., Williamson, P., Billé, R., Chalastani, V. I., Gates, R. D., Irisson, J.-O., Middelburg, J. J., Pörtner, H.-O., and Rau, G. H.: Ocean solutions to address climate change and its effects on marine ecosystems, Front. Mar. Sci., 5, 337, https://doi.org/10.3389/fmars.2018.00337, 2018.
Gattuso, J.-P., Williamson, P., Duarte, C., and Magnan, A. K.: The potential for ocean-based climate action: negative emissions technologies and beyond, Front. Climate, 2, 575716, https://doi.org/10.3389/fclim.2020.575716, 2021.
Geden, O. and Löschel, A.: Define limits for temperature overshoot targets, Nat. Geosci., 10, 881–882, 2017.
Gehlen, M., Bopp, L., and Aumont, O.: Short-term dissolution response of pelagic carbonate sediments to the invasion of anthropogenic CO2: A model study, Geochem. Geophy. Geosy., 9, Q02012, https://doi.org/10.1029/2007GC001756, 2008.
GESAMP: High level review of a wide range of proposed marine geoengineering techniques, edited by: Boyd, P. W. and Vivian, C. M. G., IMO/FAO/UNESCO-IOC/UNIDO/WMO/IAEA/UN/UN Environment/UNDP/ISA Joint Group of Experts on the Scientific Aspects of Marine Environmental Protection, Rep. Stud. GESAMP WG 41, Reports & Studies Series, ISSN 1020-4873, 2019.
Grassi, G., Stehfest, E., Rogelj, J., van Vuuren, D., Cescatti, A., House, J., Nabuurs, G.-J., Rossi, S., Alkama, R., Vinas, R. A., Calvin, K., Ceccherini, G., Federici, S., Fujimori, S., Gusti, M., Hasegawa, T., Havlik, P., Humpenöder, F., Korosuo, A., Perugini, L., Tubiello, F. N., and Popp, A.: Critical adjustment of land mitigation pathways for assessing countries' climate progress, Nat. Clim. Change, 11, 425–434, https://doi.org/10.1038/s41558-021-01033-6, 2021.
Hartmann, J., Suitner, N., Lim, C., Schneider, J., Marín-Samper, L., Arístegui, J., Renforth, P., Taucher, J., and Riebesell, U.: Stability of alkalinity in ocean alkalinity enhancement (OAE) approaches – consequences for durability of CO2 storage, Biogeosciences, 20, 781–802, https://doi.org/10.5194/bg-20-781-2023, 2023.
Harvey, L. D. D.: Mitigating the atmospheric CO2 increase and ocean acidification by adding limestone powder to upwelling regions, J. Geophys. Res.-Oceans, 113, C04028, https://doi.org/10.1029/2002GL016224, 2008.
Hauck, J., Köhler, P., Wolf-Gladrow, D. A., and Völker, C.: Iron fertilisation and century-scale effects of open ocean dissolution of olivine in a simulated CO2 removal experiment, Environ. Res. Lett., 11, 024007, https://doi.org/10.1088/1748-9326/11/2/024007, 2016.
He, J. and Tyka, M. D.: Limits and CO2 equilibration of near-coast alkalinity enhancement, Biogeosciences, 20, 27–43, https://doi.org/10.5194/bg-20-27-2023, 2023.
Ho, D. T., Bopp, L., Palter, J. B., Long, M. C., Boyd, P. W., Neukermans, G., and Bach, L. T.: Monitoring, reporting, and verification for ocean alkalinity enhancement, in: Guide to Best Practices in Ocean Alkalinity Enhancement Research, edited by: Oschlies, A., Stevenson, A., Bach, L. T., Fennel, K., Rickaby, R. E. M., Satterfield, T., Webb, R., and Gattuso, J.-P., Copernicus Publications, State Planet, 2-oae2023, 12, https://doi.org/10.5194/sp-2-oae2023-12-2023, 2023.
Ilyina, T., Wolf-Gladrow, D. A., Munhoven, G., and Heinze, C.: Assessing the potential of calcium-based artificial ocean alkalinization to mitigate rising atmospheric CO2 and ocean acidification, Geophys. Res. Lett., 40, 5909–5914, https://doi.org/10.1002/2013GL057981, 2013.
IPCC: Climate Change 2022: Mitigation of Climate Change. Contribution of Working Group III to the Sixth Assessment Report of the Intergovernmental Panel on Climate Change, edited by: Shukla, P. R., Skea, J., Slade, R., Al Khourdajie, A., van Diemen, R., McCollum, D., Pathak, M., Some, S., Vyas, P., Fradera, R., Belkacemi, M., Hasija, A., Lisboa, G., Luz, S., and Malley, J., Cambridge University Press, Cambridge, UK and New York, NY, USA, https://doi.org/10.1017/9781009157926, 2022.
IRENA: Renewable capacity statistics 2021, International Renewable Energy Agency (IRENA), Abu Dhabi, https://www.irena.org/-/media/Files/IRENA/Agency/Publication/2021/Apr/IRENA_RE_Capacity_Statistics_2021.pdf (last access: 26 October 2023), 2021.
Jones, D. C., Ito, T., Takano, Y., and Hsu, W.-C.: Spatial and seasonal variability of the air-sea equilibration timescale of carbon dioxide, Global Biogeochem. Cy., 28, 1163–1178, 2014.
Keller, D. P., Feng, E. Y., and Oschlies, A.: Potential climate engineering effectiveness and side effects during a high carbon dioxide-emission scenario, Nat. Commun., 5, 3304, https://doi.org/10.1038/ncomms4304, 2014.
Kheshgi, H. S.: Sequestering atmospheric carbon dioxide by increasing ocean alkalinity, Energy, 20, 915–922, 1995.
Köhler, P., Abrams, J. F., Völker, C., Hauck, J., and Wolf-Gladrow, D. A.: Geoengineering impact of open ocean dissolution of olivine on atmospheric CO2, surface ocean pH and marine biology, surface ocean pH and marine biology, Environ. Res. Lett., 8, 014009, https://doi.org/10.1088/1748-9326/8/1/014009, 2013.
Lawrence, M. G., Schäfer, S., Muri, H., Scott, V., Oschlies, A., Vaughan, N. E., Boucher, O., Schmidt, H., Haywood, J., and Scheffran, J.: Evaluating climate geoengineering proposals in the context of the Paris Agreement temperature goals, Nat. Commun., 9, 3734, https://doi.org/10.1038/s41467-018-05938-3, 2018.
Lund, J. F., Markusson, N., Carton, W., and Buck, H. J.: Net zero and the unexplored politics of residual emissions, Energy Research & Social Science, 98, 103035, https://doi.org/10.1016/j.erss.2023.103035, 2023.
Mant, R. C., Jones, D. L., Reynolds, B., Ormerod, S. J., and Pullin, A. S.: A systematic review of the effectiveness of liming to mitigate impacts of river acidification on fish and macro-invertebrates, Environ. Pollut., 179, 285–293, 2013.
Matthews, H. D., Gillett, N. P., Stott, P. A., and Zickfeld, K.: The proportionality of global warming to cumulative carbon emissions, Nature, 459, 829–832, 2009.
Moras, C. A., Bach, L. T., Cyronak, T., Joannes-Boyau, R., and Schulz, K. G.: Ocean alkalinity enhancement – avoiding runaway CaCO3 precipitation during quick and hydrated lime dissolution, Biogeosciences, 19, 3537–3557, https://doi.org/10.5194/bg-19-3537-2022, 2022.
National Academies of Sciences, Engineering, and Medicine (NASEM): A Research Strategy for Ocean-based Carbon Dioxide Removal and Sequestration, The National Academies Press, Washington, D.C., https://doi.org/10.17226/26278, 2021.
Nawaz, S., Peterson St-Laurent, G., and Satterfield, T.: Public evaluations of four approaches to ocean-based carbon dioxide removal, Clim. Policy, 23, 379–394, 2023.
Renforth, P.: The negative emission potential of alkaline materials, Nat. Commun., 10, 1401, https://doi.org/10.1038/s41467-019-09475-5, 2019.
Renforth, P. and Henderson, G.: Assessing ocean alkalinity for carbon sequestration, Rev. Geophys., 55, 636–674, https://doi.org/10.1002/2016RG000533, 2017.
Riebesell, U., Fabry, V. J., Hansson, L., and Gattuso, J.-P.: Guide to Best Practices in Ocean Acidification Research and Data Reporting, Publications Office of the European Union, 260 pp., https://doi.org/10.2777/66906, 2010.
Smith, S. M., Geden, O., Nemet, G. F., Gidden, M. J., Lamb, W. F., Powis, C., Bellamy, R., Callaghan, M. W., Cowie, A., Cox, E., Fuss, S., Gasser, T., Grassi, G., Greene, J., Lück, S., Mohan, A., Müller-Hansen, F., Peters, G. P., Pratama, Y., Repke, T., Riahi, K., Schenuit, F., Steinhauser, J., Strefler, J., Valenzuela, J. M., and Minx, J. C.: The state of carbon dioxide removal – 1st edition, Technical report, OSF, https://doi.org/10.17605/OSF.IO/W3B4Z, 2023.
Steenkamp, R. C. and Webb, R.: Legal considerations relevant to research on ocean alkalinity enhancement, in: Guide to Best Practices in Ocean Alkalinity Enhancement Research, edited by: Oschlies, A., Stevenson, A., Bach, L. T., Fennel, K., Rickaby, R. E. M., Satterfield, T., Webb, R., and Gattuso, J.-P., Copernicus Publications, State Planet, 2-oae2023, 10, https://doi.org/10.5194/sp-2-oae2023-10-2023, 2023.
UNFCCC: The Paris Agreement (FCCC/CP/2015/L.9/Rev.1), United Nations, https://unfccc.int/process-and-meetings/the-paris-agreement/the-paris-agreement (last access: 25 October 2023), 2015.
United Nations Environment Programme: Emissions Gap Report 2022: The Closing Window – Climate crisis calls for rapid transformation of societies, Nairobi, UNEP, https://www.unep.org/emissions-gap-report-2022 (last access: 23 October 2023), 2022.
Wang, H., Pilcher, D. J., Kearney, K. A., Cross, J. N., Shugart, O. M., Eisaman, M. D., and Carter, B. R.: Simulated impact of ocean alkalinity enhancement on atmospheric CO2 removal in the Bering Sea, Earth's Future, 11, e2022EF002816, https://doi.org/10.1029/2022EF002816, 2023.
- Abstract
- Climate goals and the need for carbon dioxide removal
- CDR approaches and the role the ocean could play
- Ocean alkalinity enhancement
- Motivation for developing a best practices guide
- Development of this best practice guide
- Data availability
- Author contributions
- Competing interests
- Disclaimer
- Acknowledgements
- Financial support
- Review statement
- References
- Abstract
- Climate goals and the need for carbon dioxide removal
- CDR approaches and the role the ocean could play
- Ocean alkalinity enhancement
- Motivation for developing a best practices guide
- Development of this best practice guide
- Data availability
- Author contributions
- Competing interests
- Disclaimer
- Acknowledgements
- Financial support
- Review statement
- References